the Creative Commons Attribution 4.0 License.
the Creative Commons Attribution 4.0 License.
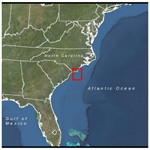
Optimizing offshore wind export cable routing using GIS-based environmental heat maps
Joni Thomas Backstrom
Nicholas Mark Warden
Colleen Marie Walsh
In the United States, there are plans to produce up to 30 GW of offshore wind power by the year 2030, resulting in numerous seabed lease areas which are currently going through the leasing or construction and operations phase. A key challenge associated with offshore wind is optimal routing and installation of the subsea power cables, which transmit power from the main offshore wind energy production area to a land-based station, where it connects to the electrical grid. By traversing a vast extent of the seafloor, the installation and operational phases of subsea power cables have the potential to result in a range of environmental impacts, which may negatively affect sensitive biological, physical, human and/or cultural resource receptors. Presented here is a case study from southeastern North Carolina to identify optimal seabed cable routes and coastal landfalls for a recently leased offshore wind farm by using a combination of publicly available data, coupled with standard environmental impact assessment methodologies and geographic information system (GIS)-based heat maps. The study identified a range of high-risk areas, in addition to a number of potential low-risk routes and landfall areas which minimize seabed user conflicts and impacts on environmentally sensitive locations. Although additional high-resolution and site-specific environmental, geological and biological surveys are required to develop a robust cable installation plan, the preliminary steps from this research optimize early-phase marine spatial planning for offshore wind projects and other similar subsea industries.
- Article
(8851 KB) - Full-text XML
-
Supplement
(526 KB) - BibTeX
- EndNote
Offshore wind is predicted to grow exponentially across the United States, with a target of 30 GW of power by the year 2030 (Energy, 2021). Off coastal North Carolina, which forms the focus of this study, the state has an ambitious wind energy goal of 2.8 GW of offshore wind energy capacity by the year 2030, which is set by the state's current renewable energy targets and based on Executive Order No. 218 (NC DEQ, 2023). A key challenge associated with offshore wind includes optimal routing and installation of the subsea power (export) cables, which transmit power from the main offshore wind energy area to a land-based station, where they connect to the electrical grid. By traversing a vast extent of the seafloor, the installation, operational and decommissioning phases of subsea power cables have the potential to result in a range of environmental impacts, which may negatively affect sensitive biological, physical, human and/or cultural resource receptors (OSPAR, 2012; BOEM, 2023a).
Several studies, especially from Europe, have assessed potential effects of export cables on a range of abiotic and biotic marine environments (Worzyk, 2009; Hammar et al., 2014; MMO, 2014; English et al., 2017; Taormina et al., 2018). During the initial export cable installation burial and operational phase, a number of different effects are generated, which include noise, electromagnetic fields (EMFs), thermal effects and increases in turbidity. Acoustic disturbances have the potential to alter the behaviour of surrounding organisms, but studies have generally shown a low probability of inflicting bodily harm or mortality on nearly all taxa that have been observed (Nedwell et al., 2012; Mooney et al., 2020). During the operational power transmission phase, EMFs, in addition to thermal effects, emitted by the export cable also have the potential to impact marine life (Gill, 2005; Snyder et al., 2019; Hutchinson et al., 2020). Taormina et al. (2018) identified EMF effects as being a key knowledge gap in offshore wind development. Initial research has shown that benthic species exhibit mixed responses to EMFs in lab settings, though behaviour alteration is a consistent outcome (Albert et al., 2020). Cumulative impact assessments have also determined the impacts of EMFs to be minor compared to other potential effects associated with subsea power cables (Bergstrom et al., 2014; Copping et al., 2020). Proper cable burial, ideally 1–2 m beneath the seafloor, is known to reduce transmission-related EMF effects, in addition to associated thermal effects (Snyder et al., 2019). Where cable burial is not possible due to substrate type, the placement of mattressing, boulders or concrete is a common approach (OSPAR, 2008; BOEM, 2023a).
Direct alteration of the seabed and increased suspended sediments are known physical effects attributed to export cable installation (Taormina et al., 2018). These physical changes have the potential to directly and indirectly affect various organisms in the surrounding ecosystem due to the disturbance (Gill, 2005; English et al., 2017). Suspended sediments generated from jetting, trenching and/or ploughing during cable installation are known to degrade water quality, result in increased turbidity, and may also lead to lethal organism burial and/or associated sub-lethal responses, with potential impacts on surrounding benthic and aquatic organisms (Worzyk, 2009; Bergstrom et al., 2014; Methratta et al., 2021). Increased suspended sediment effects are largely localized, as long-term effects are diminished with increasing distance from the altered seabed areas (Methratta, 2021; English et al., 2017; MMO, 2014). Habitat alteration from stabilizing structures placed on the seabed to reduce cable mobility is known to have a generally positive effect on benthic communities as well as fish populations (Taormina et al., 2017; Perry and Heyman, 2020). The positive socio-economic impacts associated with offshore wind, for example attraction of sessile, benthic and fish species via the artificial reef effect, are evident in the eastern United States, both within recreational fishery circles and among other ocean users (Voss et al., 2013; Smythe et al., 2021). Positive socio-economic impacts include, for example, increased coastal and marine ecotourism, new recreational diving opportunities, and improved fish stocks and fishing grounds in and around the offshore wind farm (OWF). However, some angler and fisher concerns include displacement and over-crowding, although the views of anglers are mostly positive (Voss et al., 2013; Smythe et al., 2021).
In addition to environmental impacts, submarine hazards pose another challenge for managing risks associated with export cables. Monitoring conducted for other OWF sites, predominantly in northern Europe, has revealed some of the potential effects, and possible mitigation practices, for export cable route planning and installation in a case study that examined dozens of OWF's cable exposure and free span due to scour or improper burial that occurred at some offshore wind locations (English et al., 2017). Research has shown that avoidance of submarine geohazards (e.g. steep seabed areas, earthquake-prone locations) is critical for cable route selection and infrastructure protection (Carter et al., 2014). Cumulative impact assessments – to reduce potential impacts attributed to other marine activities – using geospatial techniques is a common approach in offshore wind impact studies (Carter et al., 2014; Lonsdale et al., 2020; Hammar et al., 2020; Choi et al., 2021; Gusatu et al., 2021). Marine spatial planning, by identifying a number of different sea and seabed users within a confined spatial context using geographic information system (GIS) techniques and impact assessments, is critical for successful and sustainable OWF development.
The main aim of this study is to explore the environmental impacts associated with subsea power cable installation and transmission and to propose monitoring and mitigation techniques to guide optimal subsea power cable routing. This is accomplished using an innovative combination of desktop studies of publicly available data, coupled with standard environmental impact assessment (EIA) methodologies and GIS-based geospatial and modelling methods for a proposed OWF off the coast of southeastern North Carolina. The ultimate aim is to optimize marine spatial planning, minimize ecological impacts, and assist with critical coastal and offshore management decision-making during the early phases of OWF development.
The recently leased Carolina Long Bay offshore wind farm, located off the coast of southeastern North Carolina, forms the focus of this study (Fig. 1). The Bureau of Ocean Energy Management (BOEM) auctioned off the Carolina Long Bay wind energy area (WEA) to prospective developers on 11 May 2022. The OWF comprises a 445 km2 offshore region located approximately 31.5 km (17 nautical miles) offshore Bald Head Island, near the terminus of the Cape Fear River. The proposed OWF is expected to generate 1.3 GW of electricity, providing more than half a million households with power, creating thousands of local jobs and generating hundreds of millions of dollars in economic revenue. The wind farm will require one or more export (power) cables, connecting the wind turbine substation to a shore-based location for subsequent energy distribution to land-based sources (NCTPC, 2021).
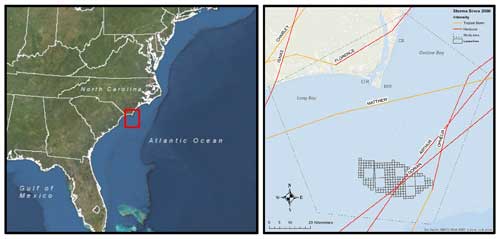
Figure 1Study area. The proposed Carolina Long Bay offshore wind farm is located in the northern Atlantic Ocean off southeast North Carolina, approximately 31.5 km south of Bald Head Island (BHI). Also shown are recent hurricane tracks that have impacted the area since 2000 (right). CFR refers to the Cape Fear River mouth (also the location of Jay Bird Shoals), and CB refers to Carolina Beach. Hurricane track data are obtained from NOAA. Satellite image (left) courtesy of © Google Maps.
For this study, the export cable “area of interest” extends from Masonboro Island in the northeast to the South Carolina border in the west and from the coast to as far as 40 km offshore (Fig. 1). Coastal southeastern North Carolina comprises a number of developed and undeveloped north–south- and east–west-oriented transgressive barrier islands, separated by tidal inlets (Cleary et al., 2001; Shiflett and Backstrom, 2023). Tourism is a major source of income for the rapidly growing coastal towns. Tides are semidiurnal and microtidal, with a mean tidal range of 1.37 m off Bald Head Island (NOAA, 2023). A 10-year record of wave data obtained from Station 41108, Wilmington Harbor, North Carolina (NDBC, 2024), reveals significant wave heights (Hs) are approximately 1.0 m with an average dominant wave period (Td) of 7 s; the mean wave direction is from the SSE (153°). According to the National Renewable Energy Laboratory, average offshore wind speeds at 90 m elevation within the study area range between 7.5 m s−1 at the coast to 9.0 m s−1 at the proposed wind energy area (NREL, 2023). As a result, average offshore wind speeds in North Carolina are among the most optimal along the eastern United States, and a relatively wide and shallow continental shelf makes it an ideal location for OWF development.
Southeast North Carolina lies directly in the path of North Atlantic tropical systems, creating a possible hazard for OWF development, and has been impacted by numerous hurricanes, most recently by Isaias (Cat 1 – 2020), Dorian (Cat 1 – 2019), Florence (Cat 1 – 2018) and Matthew (Cat 1 – 2016) (Fig. 1, right). Other destructive hurricanes include Bonnie and Fran in 1995 and Category 4 Hazel in 1954. The offshore seabed region comprises numerous Plio-Pleistocene high- to low-relief carbonate hard-bottom reefs, often overlain with a thin veneer of sand and gravel (Cleary et al., 1996; Backstrom, 2002). Thick regionally expansive sand bodies are not common off southeastern North Carolina and are mostly limited to either localized ancestral paleo-river channel systems or large sand shoals located adjacent to and offshore of the Cape Fear River (Cleary, 1999; Riggs et al., 1995; Backstrom, 2002).
The area eligible for turbine placement covers slightly more than 445 km2, with an expected capacity of up to 1.3 GW of wind energy. Carolina Long Bay comprises BOEM's offshore wind lease areas OCS-A 0545 and OCS-A 0546 on the Atlantic Outer Continental Shelf, which were awarded to TotalEnergies Renewables USA, LLC, and Duke Energy Renewables Wind, LLC, respectively. This area of southeast North Carolina has a range of protected species, coastal conservation areas, offshore reefs and historical shipwrecks. Additionally, the region is highly susceptible to tropical storms and hurricanes, has limited offshore sediment (apart from Frying Pan Shoals), is an important hub for both fisheries and shipping, and supports a multi-million-dollar tourism industry. The spatial arrangement and distribution of these multiple overlapping receptors across the study area make the routing, installation and operation of subsea power cables particularly challenging.
In order to quantify optimum routing and spatial mitigation measures for the export cable, this study uses a three-phased approach: (i) a desktop study and literature review of publicly available coastal and ocean data for the region and study area, (ii) a quantification of environmental sensitivity and risk based on standard EIA procedures, and (iii) the use of GIS-based spatial and modelling techniques to optimize route planning by combining heat maps and Euclidean distance tools. Temporal and spatial mitigation techniques are also incorporated into the assessment.
The primary strategy in evaluating optimum cable routing for Carolina Long Bay was by means of an environmental heat map, in essence mapping the intensity of different receptors based on sensitivity (e.g. reefs, protected areas) and areal extent or, conversely, assessing potential impacts on the integrity of the export cables (e.g. shipping and navigation areas). Before generating the heat maps, a thorough review of publicly available BOEM reports, relevant research, published papers, grey literature and other desktop studies was conducted. This allowed for a strategy development for the heat map exercise and the choice of relevant data to use in the operations, while also carefully considering all types of data available (Table 1). Sensitivity values and included datasets in the analysis were determined based on standard EIA methodology, local knowledge and the authors' best judgement. There is naturally some subjectivity to this, and different values and datasets will undoubtedly result in different outcomes. However, it is important to note that this is a “high-level exercise” that can be adopted by other OWF scientists and managers who may use different values and datasets based on local environmental knowledge, site-specific stakeholder concerns, government regulations and liaison with topic-specific experts.
Environmental review processes for permitting and developing OWFs consider a wide range of factors, including stakeholder consultation, scoping and environmental impact assessments (BOEM, 2023a). To minimize potential impacts from any proposed development, a variety of mitigation measures is required. Depending on the type of impact, often either spatial or temporal mitigation is chosen as the most appropriate option. Certain highly mobile receptors were displayed in the map figures but were excluded from the spatial analysis. For these receptors, the most appropriate management measure does not involve spatial mitigation. Possible temporal mitigation measures or other mitigation strategies for these receptors are discussed in the Results section.
The spatial analysis to produce the heat map follows a basic workflow that was replicated for each GIS layer and performed using ESRI's ArcMap 10.8.2, which highlights corridors exhibiting maximum distances from identified hazards. Each layer that was included in the analysis was imported as a shapefile, which contained vector data within the GIS interface (Table 1). The input layers were subsequently converted into individual raster files, with a 100 m spatial resolution. A risk value was assigned to each fixed (i.e. non-mobile) spatial layer, based on the anticipated impact from the installation of the export subsea cable on each receptor or as a hazard to cable-laying operations. Risk values were not assigned to highly mobile receptors (e.g. whale migration patterns, essential fish habitat (EFH), hurricanes) due to their temporary presence. Assigning risk values to sensitive receptors based on anticipated impacts is a practice that has been used in offshore wind planning before and is a common approach in environmental impact assessments (Bergstrom et al., 2014; BOEM, 2023a). Marine spatial planning also incorporates many of the design components of our study, primarily by identifying suitable areas for specified uses in the marine environment through minimization of conflict (Lonsdale et al., 2020; Choi et al., 2021).
Risk values for our model ranged from one to three (Table 2). A value of “1” was assigned to areas that, without proper avoidance, could lead to minor impacts on receptors, such as temporary area closures or obstacles to other ocean users. For areas where cable construction is expected to lead to major impacts on receptors, either human or ecological, a value of “2” was assigned. Lastly, a value of “3” was given to any areas that represent a major hazard or threat to the integrity of the cable structure itself or cable-laying equipment or for regions under the jurisdiction of policies which disallow such infrastructure. These areas must be “avoided at all costs”, and in most cases, a sufficient spatial buffer/exclusion zone around the hazard must be adhered to. The following tables identify each of the GIS layers that were used as inputs and their associated risk values which were retained in the model (Tables 1 and 2).
Table 1Data sources and description, including recommended mitigation type, for each GIS layer used in the case study. Specific data source links are provided in Table S1 in the Supplement.
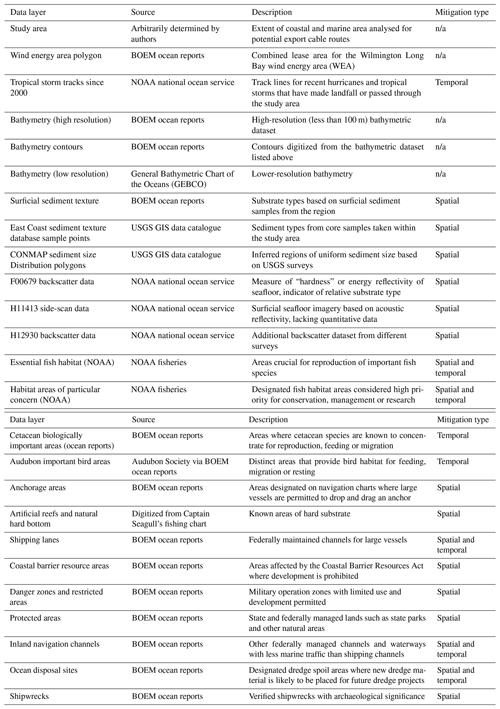
Table 2Associated risk value for each layer/sensitive receptor included in the spatial model. The value was based on standard Environmental Impact Assessment and National Environmental Policy Act (EIA/NEPA) policy, local environmental knowledge and the authors' best judgement. Mobile species (e.g. whales and sharks, EFH) and other temporal mitigation layers are not included.
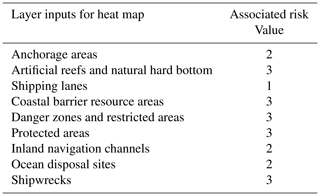
The following are risk value keys used in the study:
-
Without avoiding this area, minor impacts are expected (shipping lanes).
-
Without avoiding this area, major impacts are expected (anchorage areas, ocean disposal sites, navigation channels).
-
This area must be avoided entirely. It contains hazards with the potential to compromise equipment or the cable itself, and regulations prevent cable-laying operations in this area (danger zones/restricted areas, protected areas, shipwrecks/artificial reefs).
Risk values for fixed/spatially defined receptors were incorporated into the raster file for each layer by means of the reclassify tool in ArcMap, which allowed each raster layer to have the risk level populated as its cell value for each cell in the raster. These individual files were subsequently combined into one raster layer covering the entire extent of the study area using the raster calculator tool. A blank raster with identical cell sizes and a value of 0 for each cell was added to the operation to function as a background, allowing the output to cover the entire extent of the study area. The output from this tool created a composite heat grid, displaying a value for each cell that represented the total risk associated with that space. Cells that did not contain any hazards or did not overlap with any receptors retained a value of 0, indicating no environmental risk. The heat grid was symbolized according to cell value, highlighting differences in risks associated with export cable operations across the entire study area.
The composite heat grid was used as an input for the Euclidean distance tool to reveal microregions within the study area likely to exhibit minimized impacts on the cable and surrounding environment. With a broad understanding of the distribution of risk factors within the export cable region, possible export cable corridors were digitized according to the maximum distance from surrounding hazards and sensitive receptors.
4.1 Physical environment
The initial results of the case study reveal several key marine environmental characteristics in which the proposed offshore wind project is set to take place. With the northernmost extent of the WEA located 31.5 km south of the nearest shoreline on Bald Head Island, a substantial area of seabed must be assessed in order to install the export cable. One of the most prominent features of this offshore area is Frying Pan Shoals, a 45 km stretch of sand waves and shallow shoals that measure as little as 3 m deep in some offshore areas between the WEA and the closest onshore location (Fig. 2 – left). Water depths across the region range from less than 10 m nearshore and along the shoals up to more than 30 m offshore.
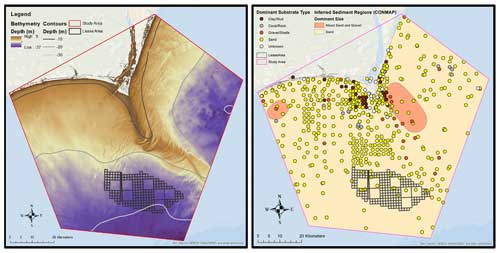
Figure 2Water depths (left) and seabed sediment distribution (right) within the study area. Note the extensive sand shoals extending towards the southeast, approximately between the 10 and 20 m contours, associated with Frying Pan Shoals. The majority of the seabed surficial sediment is comprised of sand, with some smaller areas of mud, gravel, shell and rock. Sources: NOAA (bathymetry) and USGS CONMAP (sediments).
The bathymetric data and contours (Fig. 2 – left) clearly show the extent of Frying Pan Shoals (extending towards the southeast between the 10 and 20 m contours), as well as some smaller sand wave fields to the north of the WEA, on the western side of the shoals. Assuming the shallowest of these features are avoided during cable-laying operations, most of the seafloor area that must be covered by the cable falls between 10 and 20 m depths, which is easily manageable by cable installation companies. Bathymetry data are particularly useful in determining the equipment type and installation methods for the export cable.
According to the USGS surface sediment database, the seabed sediments within the study area are primarily composed of sand (yellow), although shells and mud (green), in addition to rock and gravel (orange), are also present (Fig. 2 – right). A good understanding of sediment cover is especially important when it comes to cable installation methods, installation vessel anchoring/mooring methods and general benthic characteristics. The presence of natural hard-bottom reefs (likely identified as rock sediment) throughout the study area is well known and is an important factor to consider in cable routing. Hard bottom, also characterized as essential fish habitat, is discussed separately under “Biological resources”.
Previous seabed surveys within Long Bay in the late 1990s, which included extensive side-scan sonar, vibracores and surface sediment sampling, revealed that the shoreface was not a viable source of long-term compatible beach nourishment sand, instead mostly comprising a mix of thin, muddy sediment layers or hard-bottom outcrops (Cleary et al., 2001). The only well-known thick sand bodies include Jay Bird Shoals (situated at the mouth of the Cape Fear River – refer to CFR in Fig. 1) and Frying Pan Shoals, located adjacent to or offshore of the Cape Fear River inlet, respectively (Fig. 2 – left). To date, the specific outline and distribution of Frying Pan Shoals have not been contoured or determined by regulatory agencies.
4.2 Biological resources
The marine environment encompassed by the study area, including some of the coastal areas that could be impacted by the cable landfall location, serves as an important habitat for a variety of fish, birds and mammals (Taylor et al., 2016; BOEM, 2022b). Large sections of the study area are classified as essential fish habitat (EFH) by NOAA. Habitat areas of particular concern (HAPCs) are a subset of EFH that provide particularly important ecological functions or face increased vulnerability to degradation. Much of the study area consists of zones that are designated as HAPCs (Fig. 3 – left). Where possible, these areas should be avoided during cable routing and installation plans or at least discussed carefully with the management and conservation agencies in charge of protecting these habitats.
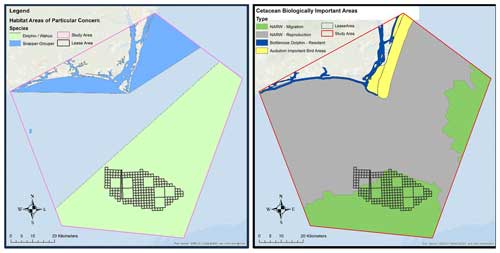
Figure 3Habitat areas of particular concern (HAPCs – left) and cetacean biologically important areas (BIAs – right). HAPCs include the dolphin/wahoo (green) and snapper/grouper fisheries (blue). BIAs include North Atlantic right whale migration and reproduction areas (green and grey, respectively), resident bottlenose dolphins (dark blue), and designated Audubon important bird areas (yellow).
The HAPCs in Fig. 3 (left) represent habitat for pelagic species, such as dolphin/wahoo fisheries, in addition to commercially important demersal fish species, such as the snapper/grouper fishery, both of which are managed by the South Atlantic Fishery Management Council (https://safmc.net/fishery-management/, last access: 23 March 2024). Parcels of essential fish habitat (i.e. hard bottom) are also dispersed throughout the study area. Not only do these areas represent an important habitat for benthic and fish species, but they also present a challenge to export cable burial capabilities due to the presence of sub-cropping and/or outcropping rock on the seabed.
In addition to fish, the study area contains habitat for other classes of marine vertebrates, most notably marine mammals, sea turtles and birds (Fig. 3 – right). Biologically important areas (BIAs) for prominent cetaceans are known to inhabit the area, especially the North Atlantic right whale (NARW) and bottlenose dolphins. Important coastal bird areas, as designated by Audubon, are also included.
In order to mitigate the effects of export cable installation on NARW migration routes, temporal mitigation (i.e. avoiding construction activities when they are known to be present in the region) is recommended. Any proposed cable route near the coast is also highly likely to overlap with bottlenose dolphin resident areas, and it has the potential to impact important bird areas if landfall is made along the shoreline north of Bald Head Island (Fig. 3 – right). Another environmental designation that is relevant to cetacean distribution is the North Atlantic right whale seasonal management area (SMA). This is a designated offshore zone that restricts the speed of large vessels during certain months of the year, when right whales are known to migrate off the coast of North Carolina (Fig. 3 – left).
4.3 Regulated areas and obstructions
Landfall location is an important aspect of the design process for export cable routing. The transition between marine and terrestrial environments presents a unique engineering challenge. Additionally, much of the shoreline within the study area is subject to prohibitive regulations, which must be planned around carefully (Fig. 4). In addition to protected natural areas, there are large tracts of land that are subject to the Coastal Barrier Resources Act (CBRA), which prohibits development and a variety of other anthropogenic activities (Fig. 4 – left). There are also restricted areas along the western shoreline of the lower Cape Fear River, classified as danger zones and strictly reserved for governmental and/or military use (Fig. 4 – left). In terms of mitigation, these restricted areas must be circumvented or avoided using spatial exclusion zones (i.e. establishing a no-go boundary) when planning the export cable route.
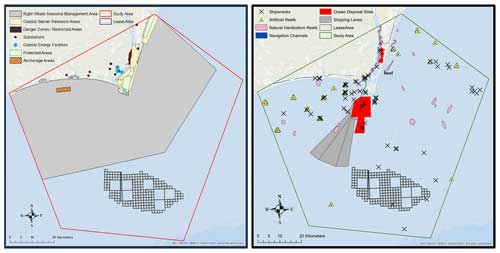
Figure 4Left: right whale seasonal management area (SMA), terrestrial protected areas, restricted areas, anchorage areas, substations and coastal energy facilities (landfall focus). Right: shipwrecks, hard-bottom reefs, artificial reefs, disposal sites, shipping lanes and inland navigation routes.
Other constraints to cable routing come in the form of physical obstructions on the seafloor (Fig. 4 – right). These include shipwrecks (many of which date back to the Civil War era, e.g. blockade runners), natural essential fish habitat hard-bottom reefs, artificial reefs and ocean disposal sites. Areas dominated by rubble or coarse gravelly sediment, often located adjacent to the hard-bottom reefs, may also present challenges to cable-laying operations.
Hard-bottom reefs are especially prevalent in the region, but due to limited seafloor mapping and the sub-cropping nature of these features, mapped and confirmed reefs undoubtedly only form a small fraction of the total area present within the region (Cleary et al., 1996, 2001; Taylor et al., 2016). Not only do these areas represent important habitat for benthic and fish species, but they also present a challenge to export cable burial. Recent Carolina Long Bay informational stakeholder workshops held by BOEM suggest that the hard-bottom areas are of significant concern for many recreational and commercial fishers, often targeted due to the presence of valuable fish stocks.
Inland navigation presents another challenge to cable routing. With the deep-water port of Wilmington located approximately 35 km up the Cape Fear River, many large vessels approach the port in the offshore area near the WEA (Fig. 4 – right). There are also anchorage areas located near the Cape Fear River Inlet which present a direct risk to the proposed export cables due to anchoring risks which may compromise structural integrity. An additional navigation and cable routing constraint is the broad footprint of the traffic separation scheme (TSS). Vessels approaching the port are required to remain within a designated shipping lane that covers a particularly large area and happens to be located between the wind farm location and several potential landfall sites. Although avoiding navigation areas is not a requirement for cable routing, running an export cable through any part of the TSS will lead to temporary area closures, which may have socio-economic impacts on the local shipping industry. Proper cable burial will be critical within these areas to prevent any kind of cable exposure or free span, which has been known to occur at some OWF sites in Europe (English et al., 2017).
4.4 Environmental heat maps
This section provides a summary and brief discussion of the GIS-based environmental heat maps, delineating high-risk versus low-risk areas for export cable routing, based on the sum of risk values for each spatially defined or fixed environmental constraint considered in the assessment. A composite heat map of physical constraints, which includes reefs, shipwrecks, shipping and navigation areas, anchorage and disposal sites, coastal protected areas, and military locations (Fig. 5), shows specific areas of environmental constraint which represent a high composite risk score. There are several areas that contain scores of 3 to 4, which in many cases represent hard-bottom reefs within the proposed export cable region and present substantial installation/burial and integrity risk for the cables. Areas with a score of 4 or higher often represent areas that contain overlapping hazard areas, especially coastal protected locations. In both cases, there is high potential for the identified hazards to hinder export cable routing, and therefore these areas should be avoided.
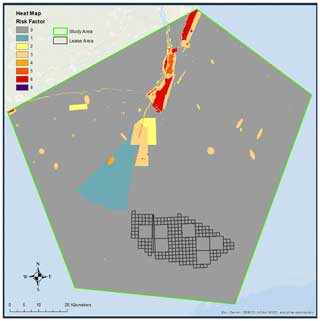
Figure 5Composite raster heat map of selected fixed constraints to export cable routing and installation. Highly migratory species (e.g. whales and sharks) and/or mobile receptors and hazards (e.g. fishing vessels, hurricanes) are not included in the figure. EFH associated with Frying Pan Shoals has also not been included in the heat map since this has not been officially delineated or defined to date based on physical or biological characteristics.
The most restricted high-risk zone, based on the combined risk value of all the hazards in the area, extends from most of Bald Head Island north towards Carolina Beach State Park. The protected areas within this region include state natural areas, coastal reserve sites, coastal barrier resource areas (CBRAs), a state park and a military operations zone. These multiple protected nature and military no-go areas may have significant implications for export cable planning and installation. By avoiding certain high-risk areas, cable re-routing will undoubtedly increase capital expenditure (estimated at approximately USD 1 million per kilometre of cable) and operating costs for the offshore wind energy developers. This is a common experience as OWF developers navigate complex environments and engage with key state, federal and regulatory stakeholders (e.g. military, fisheries, conservation agencies) early in the planning phase.
4.5 Cable route options
In addition to a standard heat map, a Euclidean distance analysis map generated in ArcGIS (Fig. 6) represents distance to potential hazards and environmentally sensitive locations. This modelling tool allows for an overview of physical constraints based on distance and helps establish possible exclusion areas around areas considered no go or high risk, which include e.g. shipwrecks, military facilities, reefs and protected areas.
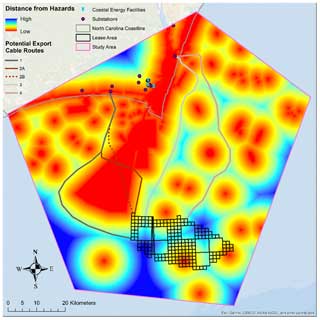
Figure 6GIS-based Euclidean distance results showing areas of minimal risk (dark blue to light blue), high-risk areas (yellow and red) and five possible export cable route options (1, 2A/B, 3 and 4).
A review of the Euclidean distance to hazards map shows five possible cable route options (Fig. 6 – options 1, 2A/B, 3 and 4) on either side of the Cape Fear River and Frying Pan Shoals. These routes were developed based on the favourable areas displayed by the hazard proximity modelling data. The results show that cable route Option 2B is the shortest distance to landfall from the WEA. However, it also interferes and crosses diagonally across much of the shipping channel and therefore should be avoided if possible. According to German marine spatial planning studies, the best option when crossing a shipping channel is to run perpendicular to it (i.e. the shortest distance) in order to avoid temporary closures during installation or cable damage due to vessel stranding and possible anchor drops (BSH, 2009, 2017). However, these scenarios are unlikely to occur, especially if proper cable burial depths are achieved.
A longer cable route crossing diagonally across the shipping channel is presented as alternative Option 2A, which follows European export cable and shipping channel guidelines. Cable route Option 1 avoids the shipping channel entirely, reducing constraints and risks, but is 40 % longer compared to Option 2B. Though there is a substantial trade-off for each of these scenarios, providing several options allows the developer to weigh the benefits and drawbacks of each scenario.
Another alternative may be installing the export cables along the eastern side of Frying Pan Shoals and Bald Head Island, parallel to the shoreline of the southern beaches of New Hanover County (cable route Option 3). With an electrical substation located in the northern half of developed Carolina Beach, the export cable could make landfall near this site while avoiding protected coastal areas. Another possibility is Option 4, a longer route which starts at the eastern half of the WEA and extends further offshore, allowing for complete or partial avoidance of Frying Pan Shoals and EFH. The landfall location would be the same as Option 3. According to NOAA, EFH and Frying Pan Shoals are not currently spatially defined and are therefore not included as a physical constraint in this modelling study, although the authors acknowledge that it is an important consideration. The EFH within the study area is primarily based on highly migratory Atlantic fish, especially sharks (NOAA, 2021). Should EFH be spatially defined around Frying Pan Shoals in the future, this would then need to be included in any similar GIS-based modelling studies.
Thick sand bodies are ideal for cable burial, which would be advantageous for the proposed export cable routes presented herein; however large mobile bedforms may result in cable free span or exposure with possible impacts on cable integrity and energy transmission (e.g. English et al., 2017). There are also scientific studies being conducted by BOEM to assess the viability of using Frying Pan Shoals as a long-term source of beach nourishment sand for local coastal communities (Pickens, 2021). This scenario needs to be assessed carefully in order to avoid any cumulative impacts (i.e. dredging for sand and cable burial) and possible conflict in terms of sand resource management.
Two potential substations present themselves as the most likely export cable landfall destinations, which is an important consideration when planning cable routes, allowing a direct connection to the electrical grid. Each substation is located on either side of the Cape Fear River mouth and is the closest in proximity to naturally presented corridors from the WEA, while also maximizing the distance from hazards along the entire route. Substations were also selected on either side of the Cape Fear River due to the dual leases awarded to energy developers.
With two companies awarded part of the lease area, planning two separate projects complicates the operation, particularly with export cable routing. The developers retain the option to run each of their export cables parallel to one another along the same corridor, eventually making landfall at the same electrical substation. Alternatively, the export cable routes could start from opposite ends of the WEA and take entirely different paths to different landfall locations several miles from one another. This second possibility seems more likely based on our examination of the layout of the region as part of the case study.
The results of this GIS-based modelling and desktop study provide important preliminary insights into the environmental characteristics of the project area and implications for export cable routing. From a high-level, early-cable-planning perspective, the results are promising. The study provides an overview of key MSP constraints, including important coastal and marine areas that should be avoided due to high risk.
Early-phase MSP, to minimize conflict with other sea and seabed users, has been an important tool used by wind developers and government since the early days of offshore wind development, particularly in the UK and western Europe (Douvere and Ehler, 2010; Berkenhagen et al., 2010; Jay, 2010; Gimpel et al., 2015) but also in the United States, e.g. offshore New York (van Haaren and Fthenakis, 2011). Many of these studies used GIS and modelling techniques to map potential conflict areas, particularly for fisheries, dredging areas, cables and pipelines, shipping and navigation areas, marine protected areas (MPAs), and military locations. Other studies, where wind farms are being planned, continue to use MSP techniques and sea-user modelling methods to identify optimum locations for offshore wind areas, including off Taiwan (Zhang et al., 2017), off Spain (Rodríguez-Rodríguez et al., 2016; Abramic et al., 2021) and in the Baltic Sea (Göke and Mohn, 2018).
Although there are numerous published papers examining the effects and ecological impacts of OWFs, there are far fewer studies which focus specifically on subsea power cables (Taormina et al., 2018). Case studies, such as this one, which combine potential export cable routes, EIA principles, MSP and GIS modelling studies for planning purposes are not readily found in the published literature. There are undoubtedly private engineering and environmental consultancy companies that use similar techniques, but these studies are not readily available, most likely due to commercial sensitivities.
In most cases, including in this study, the potential cable route corridor areas (anywhere between the WEA and the coast) often exceed the proposed wind energy area. The competing marine-user interests within this larger space between the WEA and the coast are more numerous, complex and varied (e.g. nearshore dredging and disposal sites, navigation channels, inlets and shoals, coastal MPAs, reefs and shipwrecks, coastal homeowners, important commercial and recreational fishing areas, tourism, beach erosion). In essence, this GIS-based modelling desktop study provides a relatively easy, fast and inexpensive method of identifying potential offshore wind cable corridors, which can be adopted not only by other wind developers, but also by similar marine industries like subsea telecommunications and pipelines.
It should be noted that a detailed study of each sensitive receptor that may be impacted by cable installation or operation across southeast North Carolina is beyond the scope of this study. Instead, the study should be viewed as an important high-level initial phase to identify larger ecological and physical constraints to cable routing. Following is a brief discussion of three priority environmental considerations when planning the cable route for the Carolina Long Bay wind farm. These include (i) cable burial, (ii) hard-bottom reefs/essential fish habitat and (iii) coastal landfall locations. The complex geology of southeast North Carolina's shoreface/inner shelf and the variety of sensitive coastal characteristics make these local environmental constraints especially important when planning the cable route.
5.1 Export cable corridor/burial
The original Carolina Long Bay environmental assessment (EA) by BOEM in 2015 suggested that there be at least two to three 300 m wide export cable corridors per lease area, comprising approximately 155 km of surveyed cable route. To date, no specific export cable route corridor or land-based substation connection has been established, although the leaseholder will be required to identify a corridor as part of their construction and operations plan (COP).
When it comes to detailed seabed and subsurface studies to inform export power cable routing and installation, high-resolution geophysical (e.g. multibeam bathymetry, side-scan sonar and seismic profiling) and geotechnical surveys are critical to inform final cabling design plans. They are, however, extremely expensive and time-consuming. This case study was able to procure useful information at a significantly lower cost. Importantly, the results of this study can be used to optimize survey areas for subsequent geophysical and geotechnical (G&G) data collection, especially areas of the seabed that allow for proper cable burial (i.e. at least 1–2 m of unconsolidated surface sediment). Potential export cable corridors that mitigate environmental impacts and avoid hazards and restricted areas can become the focus of subsequent G&G surveys. The data collected as part of this study will allow for fine-tuning of potential survey corridor areas, thereby reducing costs and time.
5.2 Essential fish habitat/hard bottom
The hard-bottom reefs and associated nearby features are protected under the Magnuson–Stevens Fishery Conservation and Management Act and are designated as essential fish habitat (EFH) by the National Marine Fisheries Service (NMFS). The widespread presence of hard-bottom reefs on the shoreface and inner shelf has been well documented along the sand-limited southeast coast of North Carolina (Riggs et al., 1995, 1998; Cleary et al., 1996; Backstrom, 2002). Although the prevalence of hard-bottom reefs is well known, the distribution, three-dimensional architecture, abundance and ephemeral nature of the reefs are still not well established (Riggs et al., 1995, 1998; Cleary et al., 1996; Taylor et al., 2017). Figure 7 shows a side-scan sonar image of a hard-bottom reef located off southeast North Carolina, illustrating the complexity of these environments, which can range from high-relief carbonate/limestone outcrops and rubble fields to featureless fine sand. Some estimates suggest that only 10 % of the outer continental shelf of southeast North Carolina have been mapped using standard geophysical and geotechnical survey methods, resulting in a lack of knowledge regarding the presence of these ecologically important areas (Taylor et al., 2017). The reefs comprise areas of high biodiversity, and they are known to attract and provide critical habitat to numerous fish and benthic species (Quattrini et al., 2004; Kendall et al., 2009; Freshwater et al., 2016). They are also important locations for commercial and recreational fishing and diving activities.
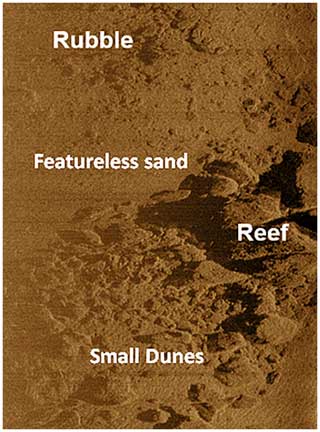
Figure 7Side-scan sonar (acoustic) image of hard-bottom reef and surroundings from southeast North Carolina. Note the mix of seabed types, including high-relief reef, rubble, small dunes/bedforms and featureless sand. Cable burial through a location like this would not be feasible due to rock presence and limited sediment thickness. The reef is located approximately 1 km offshore, south of Carolina Beach, and is referred to as reef in Fig. 4, right. Image by Joni Thomas Backstrom.
Stakeholder concerns include identifying all hard-bottom habitat within 300 m of the wind farm and associated infrastructure, including cables, in addition to mapping smaller hard-bottom units. As part of the leasing process, BOEM requires benthic habitat surveys to inform site characterization in order to assess potential environmental impacts (BOEM, 2015, 2022b). As with cable burial, high-resolution G&G surveys will be critical in identifying and mapping reef areas, where cable burial is not possible.
5.3 Export cable landfall
The coastal zone where the export cables potentially make landfall includes east-facing New Hanover County and south-facing Brunswick County beaches, separated by the Cape Fear River mouth. As a result, there are multiple environmental constraints within this area, which include e.g. important bird areas, coastally significant geological areas, EFH, shipwrecks, wetlands, critical fishery habitats and coastal barrier resource (protected) areas. Navigating these multiple inland, coastal and marine areas, coupled with identifying a likely electrical substation, presents a challenge to optimal landfall locations. A preliminary assessment from this study identified two potential landfall locations. The first location routes the export cables towards the Brunswick Nuclear Power Plant by utilizing the coolant canal that drains just offshore of Caswell Beach (Fig. 8). This landfall location avoids wetland habitats and coastal barrier resources, such as Bald Head Island Nature Conservancy and Fort Fisher State Park. The environmental impact on wetlands would be negligible to minor since the area has already been developed. Insulation methods such as a sediment cap or concrete layering would prevent cable damage due to abrasion. Additionally, tethering the cable would prevent damage. The cables' heat resistance would allow it to be submerged within the canal or buried alongside the canal route.
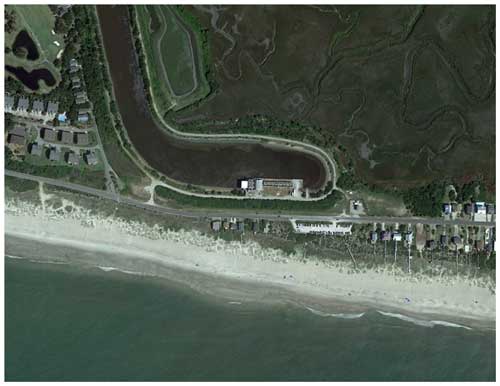
Figure 8Possible location for export cable landfall adjacent to the coolant canal of the Brunswick Nuclear Power Plant. Environmental constraints would be minimal here due to the already-developed and already-engineered nature of the location. Source: NOAA.
The second optimum landfall is located within developed central Carolina Beach, where there is an existing substation on shore. Areas further south are mostly protected coastal barrier or geologically/archeologically important areas (Bald Head Island, Zeke's Island, Fort Fisher). The developed nature of Carolina Beach would limit potential impacts on sensitive habitats like wetlands and seagrasses, which are common in the surrounding areas. There will undoubtedly be temporary socio-economic impacts in terms of beach closures and access during the short landfall construction/burial phase, but these impacts can easily be minimized by temporal mitigation techniques (e.g. avoiding construction during the busy tourist season).
Additional studies that conduct biological monitoring of potentially impacted areas will assist with the planning process. Enhanced understanding of the species present and their response to export cable installation will allow for fine-tuning of the environmental review process. Long-term monitoring is a necessary component of such studies since the export cable has the potential to permanently alter the seabed, especially if it is not removed after the decommissioning phase. For impacted ecological communities, examining their response to newly established environmental conditions associated with the cable will provide valuable insight into the long-term effects on marine ecosystems. These types of studies will improve environmental mitigation practices that can be implemented in other planned offshore wind projects and comparable subsea projects, like pipelines and telecommunications.
This GIS-based environmental modelling and mapping study has been effective in identifying environmental project constraints with easily available desktop and literature review resources. The study has identified five possible cable route corridors, with a variety of options for the OWF developers. These range from the shortest route to land from the WEA (which crosses a shipping channel) to a much longer option that avoids most environmental constraints and hazards to cable integrity. In addition, two optimal cable landfall locations, which minimize impacts on sensitive coastal receptors, have also been presented. Similar desktop studies using GIS are common for offshore wind projects, although to date studies focused exclusively on export cables and optimal routing using standard EIA methods are not readily apparent in published literature. Similar approaches have undoubtedly been used by private engineering and environmental companies, where commercial sensitivities likely ensure the resulting studies are not publicly available.
The lack of site-specific high-resolution environmental survey data may be the greatest challenge to early export cable routing and site selection. When it comes to subsea power cables, the main way to mitigate a number of potential impacts (EMF, thermal, entanglement, structural integrity) is through proper cable burial into the seabed, which is ideally at a 2 m depth for OWFs (English et al., 2008; Worzyk, 2009; OSPAR, 2012; Taormina et al., 2017; BOEM, 2023a). Once spatially defined high-risk areas have been delineated and avoided (e.g. via well-defined exclusion zones), a solid understanding of seabed surface and substrate characteristics is essential. Extensive high-resolution geophysical, geotechnical and sedimentological surveys, especially side-scan sonar, shallow seismic profiling, cone penetrometer testing and coring data, which identify seabed and sub-seabed characteristics on a much smaller scale, are required for optimal cable installation and route selection. These marine survey techniques also inform benthic characteristics and are also able to identify anthropogenic features such as shipwrecks and cables/pipelines.
The factors that are considered for environmental impact assessments pertaining to offshore wind export cables are both numerous and diverse. A wide variety of sensitive receptors exists in the offshore area between the Carolina Long Bay wind areas and the nearest shorelines that contain possible landfall sites for the export cable. While the impact on many of these receptors and export cable hazards can be minimized or avoided using spatial mitigation techniques, other receptors will require temporal mitigation to limit possible impacts. Impacts on the physical environment can generally be accounted for and mitigated spatially, while in most cases biological resources tend to have a less-defined location due to the migratory nature of many species found in the coastal and ocean environment.
Though the case study reveals clear no-go zones, there are limitations in terms of the hazards and potential impacts that cannot be revealed without additional field surveys. Arguably, there may yet be additional impacts associated with the selected cable routes that allow future surveys to focus only on the viable areas, those without known hazards or restrictions. The methods presented in this case study can be used in other OWF projects as a productive step in early-phase marine spatial planning for export cabling. The marine environment off the coast of North Carolina presents an excellent opportunity for offshore wind development; however, the sustainable use of this resource has been made a clear priority by the state and federal government. Mitigation strategies for export cables, as shown in this study, can be implemented to ensure that one of the most important components of an offshore wind operation has a minimal impact on coastal and marine resources.
Data source links are provided in Supplement Table S1.
The supplement related to this article is available online at: https://doi.org/10.5194/wes-9-1105-2024-supplement.
JTB: original study concept, literature review, data mining, writing, analysis, figure production, review, submission.
NMW: literature review, GIS analysis, modelling, data mining, writing, figure production, review.
CMW: literature review, GIS analysis, data mining, writing, figure compatibility, review. All authors have contributed to and agree with the contents of the paper.
The contact author has declared that none of the authors has any competing interests.
We certify that the submission is all original work and is not under consideration for publication in any other journal.
Publisher’s note: Copernicus Publications remains neutral with regard to jurisdictional claims made in the text, published maps, institutional affiliations, or any other geographical representation in this paper. While Copernicus Publications makes every effort to include appropriate place names, the final responsibility lies with the authors.
The authors are especially grateful to the numerous publicly available and downloadable data sources, including marine reports, the Coastal Ocean Research and Monitoring Program (CORMP – UNC Wilmington), the US Army Corps of Engineers (USACE), and the National Oceanic and Atmospheric Administration (NOAA). This study was partially funded by the Department of Environmental Sciences, UNC Wilmington. We would especially like to acknowledge the three referees who completed a thorough review of an earlier version of the manuscript, which significantly improved this version.
This paper was edited by Anca Hansen and reviewed by Silvia Paoletti and two anonymous referees.
Abramic, A., García Mendoza, G., and Haroun, R.: Introducing offshore wind energy in the sea space: Canary Islands case study developed under Maritime Spatial Planning principles, Renew. Sust. Energ. Rev., 145, 111119, https://doi.org/10.1016/j.rser.2021.111119, 2021.
Albert, L., Deschamps, F., Jolivet, A., Olivier, F., Chauvaud, L., and Chauvaud, S.: A current synthesis on the effects of electric and magnetic fields emitted by submarine power cables on invertebrates, Mar. Environ. Res., 159, 104958, https://doi.org/10.1016/j.marenvres.2020.104958, 2020.
Backstrom, J. T.: Storm-driven sedimentary changes on the shoreface of a nourished beach, Kure Beach, North Carolina, MS thesis, University of North Carolina Wilmington, United States, 49 pp., 2002.
Bergstrom, L., Kautsky, L., Malm, T., Rosenberg R., Wahlberg M., Åstrand, N., Capetillo, A., and Dan Wilhelmsson, D.: Effects of Offshore Wind Farms on Marine Wildlife – A Generalized Impact Assessment, Environ. Res. Lett., 9, 1–12, 2014.
Berkenhagen, J., Döring, R., Fock, H. O., Kloppmann, M. H. F., Pedersen, S. A., and Schulze, T.: Decision bias in marine spatial planning of offshore wind farms: Problems of singular versus cumulative assessments of economic impacts on fisheries, Mar. Policy, 34, 733–736, 2010.
BOEM: Bureau of Ocean Energy Management Commercial Wind Lease Issuance and Site Assessment Activities on the Atlantic Outer Continental Shelf Offshore North Carolina: Revised Environmental Assessment, US Department of the Interior, 105 pp., https://www.boem.gov/sites/default/files/documents/renewable-energy/state-activities/Lease Issuance Wind OCS Activities North Carolina Final Supplemental EA.pdf (last access: 28 April 2024), 2015.
BOEM: Bureau of Ocean Energy Management, Revolution Wind Farm and Revolution Export Cable – Development and Operation Biological Assessment for the U.S. Fish and Wildlife Service, 83 pp., https://www.boem.gov/sites/default/files/documents/renewable-energy/state-activities/RevWind_USFWS_BA_0.pdf (last access: 28 April 2024), 2022a.
BOEM: Bureau of Ocean Energy Management, Commercial Wind Lease Issuance and Site Assessment Activities on the Atlantic Outer Continental Shelf Offshore North Carolina: Final Supplemental Environmental Assessment, US Department of the Interior, 105 pp., https://www.boem.gov/sites/default/files/documents/renewable-energy/state-activities/Lease Issuance Wind OCS Activities North Carolina Final Supplemental EA.pdf (last access: 28 April 2024), 2022b.
BOEM: Bureau of Ocean Energy Management, National Environmental Policy Act and Offshore Renewable Energy, https://www.boem.gov/renewable-energy/national-environmental-policy-act-and-offshore-renewable-energy, (last access: 23 March 2024), 2023a.
BOEM: Bureau of Ocean Energy Management, Final Coastal Virginia Offshore Wind Commercial Project Essential Fish Habitat Assessment for the National Marine Fisheries Service, U.S. Department of the Interior, Bureau of Ocean Energy Management Office of Renewable Energy Programs, 154 pp., https://www.boem.gov/sites/default/files/documents/renewable-energy/state-activities/CVOW-C-EFH-Assessment.pdf (last access: 28 April 2024), 2023b.
BSH: Maritime spatial plan for the North Sea, German version, https://www.bsh.de/EN/TOPICS/Offshore/Maritime_spatial_planning/Maritime_Spatial_Plan_2021/maritime-spatial-plan-2021_node.html (last access: 28 April 2024), 2009.
BSH: Bundesfachplan für die deutsche ausschließliche Wirtschaftszone der Nordsee 2016/2017, https://www.bsh.de/DE/PUBLIKATIONEN/_Anlagen/Downloads/Offshore/Bundesfachplan-Nordsee/Bundesfachplan-Offshore-Nordsee-2016-2017.html, (last access: 28 April 2024), 2017.
Carter, L., Gavey, R., Talling, P., and Liu, J.: Insights into submarine geohazards from breaks in subsea telecommunications cables, Oceanography, 27, 58–67, https://doi.org/10.5670/oceanog.2014.40, 2014.
Choi, H. J., Cho, S. J., Hwang, T., Nam, J., and Hwang, C. S.: Cumulative Impact Assessment for Marine Spatial Planning: A Case Study of the Gyeonggi Bay in South Korea, J. Coastal Res., 114, 360–364, https://doi.org/10.2112/JCR-SI114-073.1, 2021.
Cleary, W. J., Riggs, S. R., Marcy, D. C., and Snyder, S. W.: The influence of inherited geological framework upon a hardbottom-dominated shoreface on a high-energy shelf: Onslow Bay, North Carolina, USA, Geological Society London Special Publications, 117, 249–266, 1996.
Cleary, W. J., McLeod, M. A., Rauscher, M. A., Johnston, M. K., and Riggs, S. R.: Beach Nourishment on Hurricane Impacted Barriers in Southeastern North Carolina, USA: Targeting Shoreface and Tidal Inlet Sand Resources, J. Coastal Res., 34, 232–255, 2001.
Copping, A., Hemery, L., Overhus, D., Garavelli, L., Freeman, M., Whiting, J., Gorton, A., Farr, H., Rose, D., and Tugade, L.: Potential Environmental Effects of Marine Renewable Energy Development – The State of Science, J. Mar. Sci. Eng., 8, 879, https://doi.org/10.3390/jmse8110879, 2020.
Douvere, F. and Ehler, C. N.: New perspectives on sea use management: Initial findings from European experience with marine spatial planning, J. Environ. Manage., 90, 77–88, 2009.
Energy: https://www.energy.gov/articles/energy-secretary-granholm-announces-ambitious-new-30gw-offshore-wind-deployment-target (last access: 24 March 2024), 2021.
English, P. A., Mason, T. I., Backstrom, J. T., Tibbles, B. J., Mackay, A. A., Smith, M. J., and Mitchell, T.: Improving Efficiencies of National Environmental Policy Act Documentation for Offshore Wind Facilities Case Studies Report, US Dept. of the Interior, Bureau of Ocean Energy Management, Office of Renewable Energy Programs, Sterling, OCS Study BOEM 2017-026, 217 pp., https://www.boem.gov/sites/default/files/environmental-stewardship/Environmental-Studies/Renewable-Energy/Improving-Efficiencies-of-National-Environmental.pdf (last access: 28 April 2024), 2017.
Freshwater, D. W., Whitfield, P. E., and Buckel, C. A.: Epibenthic community assessments indicate high spatial and temporal variability among continental shelf hard bottom sites in a marine transition zone, Reg. Stud. Mar. Sci., 5, 41–50, 2016.
Gill, A. B.: Offshore renewable energy: ecological implications of generating electricity in the coastal zone, J. Appl. Ecol., 42, 605–615, 2005.
Gimpel, A., Stelzenmüller, V., Grote, B., Bela, H., Buck, H., Floeter, J., Núñez-Riboni, I., Pogoda, B., and Temming, A.: A GIS modelling framework to evaluate marine spatial planning scenarios: Co-location of offshore wind farms and aquaculture in the German EEZ, Mar. Policy, 55, 102–115, 2015.
Göke, C., Dahl, K., and Mohn, C.: Maritime Spatial Planning supported by systematic site selection: Applying Marxan for offshore wind power in the western Baltic Sea, PLoS ONE, 13, e0194362, https://doi.org/10.1371/journal.pone.0194362, 2018.
Gusatu, L. F., Menegon, S., Depellegrin, D., Zuidema, C., Faaij, A., and Yamu, C.: Spatial and temporal analysis of cumulative environmental effects of offshore wind farms in the North Sea basin, Sci. Rep., 11, 10125, https://doi.org/10.1038/s41598-021-89537-1, 2021.
Hammar, L., Wikström, A., and Molander, S.: Assessing ecological risks of offshore wind power on Kattegat cod, Renew. Energ., 66, 414–424, 2014.
Hammar, L., Molander, S., Palsson, J., Crona, J., Carneiro, G., Johansson, T., Hume, D., Kagesten, G., Mattsson, D., Tornqvist, O., Zillen, L., Mattson, M., Bergstrom, U., Perry, D., Caldow, C., and Andersen, J.: Cumulative impact assessment for ecosystem-based marine spatial planning, Sci. Total. Environ., 734, 139024, https://doi.org/10.1016/j.scitotenv.2020.139024, 2020.
Jay, S.: Planners to the rescue: Spatial planning facilitating the development of offshore wind energy, Mar. Pollut. Bull., 60, 493–499, 2010.
Hutchinson, Z. L., Secor, D. H., and Gill, A. B.: The Interaction Between Resource Species and Electromagnetic Fields Associated with Electricity Production by Offshore Wind Farms, Oceanography, 33, 96–107, https://www.jstor.org/stable/26965753 (last access: 28 April 2024), 2020.
Kendall, M. S., Bauer, L. J., and Jeffrey, C. F. G.: Influence of Hard Bottom Morphology on Fish Assemblages of the Continental Shelf Off Georgia, Southeastern USA, Bull. Mar. Sci., 84, 265–286, 2009.
Lonsdale, J., Nicholson, R., Judd, A., Elliott, M., and Clarke, C.: A novel approach for cumulative impacts assessment for marine spatial planning, Environ. Sci. Policy, 106, 125–135, https://doi.org/10.1016/j.envsci.2020.01.011, 2020.
MMO: Marine Management Organization, UK, Review of environmental data associated with post-consent monitoring of offshore wind farms, 208 pp., https://assets.publishing.service.gov.uk/media/5a74a50fed915d0e8e399ddb/1031.pdf (last access: 24 March 2024), 2014.
Methratta, E. T.: Distance-Based Sampling Methods for Assessing the Ecological Effects of Offshore Wind Farms: Synthesis and Applications to Fisheries Resource Studies, Front. Mar. Sci., 8, https://doi.org/10.3389/fmars.2021.674594, 2021.
Mooney, T., Andersson, M., and Stanley, J.: Acoustic impacts of offshore wind energy on fishery resources, Oceanography, 33, 82–95, https://www.jstor.org/stable/10.2307/26965752 (last access: 28 April 2024), 2020.
Nedwell, J. R., Brooker, A. G., and Barham, R. J.: Assessment of underwater noise during the installation of export power cables at the Beatrice Offshore Wind Farm (E318R0106), Subacoustech Environmental, https://marine.gov.scot/datafiles/lot/BOWL/ES/ES Volume 4 - Annexs/7B OfTW Underwater Noise/Annex 7B OfTW Underwater Noise.pdf (last access: 28 April 2024), 2012.
NCDEQ: North Carolina Department of Environmental Quality, https://www.deq.nc.gov/energy-climate/offshore-wind-development (last access: 24 March 2024), 2023.
NCTPC: North Carolina Transmission Planning Collaborative, Report on the NCTPC 2020 Offshore Wind Study, http://www.nctpc.org/nctpc/document/REF/2021-06-07/2020_NCTPC_Offshore_Wind_Report_06_07_2021-FINAL.pdf (last access: 28 April 2024), 2021.
NDBC: National Data Buoy Center, https://www.ndbc.noaa.gov/station_history.php?station=41108, last access: 28 April 2024.
NOAA: https://www.habitat.noaa.gov/apps/efhmapper/?page=page_7, (last access: 28 April 2024), 2021.
NOAA: Tides and Currents. Station # 8658901, https://tidesandcurrents.noaa.gov/stationhome.html?id=8658901 (last access: 28 April 2024), 2023.
NREL: National Renewable Energy Laboratory, https://windexchange.energy.gov/maps-data, last access: 28 April 2024.
OSPAR: OSPAR Commission. Background document on potential problems associated with power cables other than those for oil and gas activities, 50 pp., https://www.ospar.org/documents?v=7128 (last access: 28 April 2024), 2008.
OSPAR: Guidelines on Best Environmental Practice (BEP) in Cable Laying and Operation. OSPAR Commission, 18 pp., https://www.gc.noaa.gov/documents/2017/12-02e_agreement_cables_guidelines.pdf (last access: 28 April 2024), 2012.
Perry, R. L. and Heyman, W. D.: Considerations for offshore wind energy development effects on fish and fisheries in the United States, Oceanography, 33, 28–37, https://www.jstor.org/stable/10.2307/26965747 (last access: 28 April 2024), 2020.
Pickens, B. A.: Assessment of Frying Pan Shoals as a potential sand source in the Cape Fear Region of North Carolina, Sterling (VA), US Department of the Interior, Bureau of Ocean Energy Management, OCS Study BOEM 2021-028, 81 pp., https://espis.boem.gov/final reports/BOEM_2021-028.pdf (last access: 28 April 2024), 2021.
Quattrini, A. M., Ross, S. W., Sulak, K. J., Necaise, A. M., Casazza, T. L., and Dennis, G. D.: Marine fishes new to continental United States waters, North Carolina, and the Gulf of Mexico, Southeast. Nat., 3, 155–172, 2004.
Rodríguez-Rodríguez, D., Abdul Malak, D., Soukissian, T., and Sánchez-Espinosa, A.: Achieving Blue Growth through maritime spatial planning: Offshore wind energy optimization and biodiversity conservation in Spain, Mar. Policy, 73, 8–14, https://doi.org/10.1016/j.marpol.2016.07.022, 2016.
Riggs, S. R., Cleary, W. J., and Snyder, S. W.: Influence of inherited geologic framework on barrier shoreface morphology and dynamics, Mar. Geol., 126, 213–234, 1995.
Riggs, S., Ambrose, W., and Cook, J.: Sediment production on sediment-starved continental margins: the interrelationship between hardbottoms, sedimentological and benthic community processes, and storm dynamics, J. Sediment Res., 68, 155–168, 1998.
Shiflett, S. A. and Backstrom, J. T.: Impacts of Hurricane Isaias (2020) on geomorphology and vegetation communities of natural and planted dunes in North Carolina, J. Coastal Res., 39, 587–609, 2023.
Smythe, T., Bidwell, D., and Tyler, G.: Optimistic with reservations: The impacts of the United States' first offshore wind farm on the recreational fishing experience, Mar. Policy, 127, 10440, https://doi.org/10.1016/j.marpol.2021.104440, 2021.
Snyder, D. B., Bailey, W. H., Palmquist, K., Cotts, B. R. T., and Olsen, K. R.: Evaluation of Potential EMF Effects on Fish Species of Commercial or Recreational Fishing Importance in Southern New England, OCS Study BOEM, 2019-049, https://espis.boem.gov/final reports/BOEM_2019-049.pdf (last access: 3 May 2024), 2019.
Taormina, B., Bald, J., Want, A., Thouzeau, G., Lejart, M., Desroy, N., and Carlier, A.: A review of potential impacts of submarine power cables on the marine environment: Knowledge gaps, recommendations and future directions, Renew. Sust. Energ. Rev., 96, 380–391, https://doi.org/10.1016/j.rser.2018.07.026, 2018.
Taylor, J. C., Paxton, A. B., Voss, C. M., Sumners, B., Buckel, C. A., Vander Pluym, J., Ebert, E. B., Viehman, T. S., Fegley, S. R., Pickering, E. A., Adler, A. M., Freeman, C., and Peterson, C. H.: Benthic Habitat Mapping and Assessment in the Wilmington-East Wind Energy Call Area, OCS Study BOEM 2016-003 and NOAA Technical Memorandum 196, https://coastalscience.noaa.gov/data_reports/benthic-habitat-mapping-and-assessment-in-the-wilmington-east-wind-energy-call-area-final-report/ (last access: 3 May 2024), 2016.
van Haaren, R. and Fthenakis, V.: GIS-based wind farm site selection using spatial multi-criteria analysis (SMCA): Evaluating the case for New York State, Renew. Sust. Energ. Rev., 15, 3332–3340, 2011.
Voss, C. M., Peterson, C. H., and Fegley, S. R.: Fishing, Diving, and Ecotourism Stakeholder Uses and Habitat Information for North Carolina Wind Energy Call Areas, U.S. Department of the Interior, Bureau of Ocean Energy Management, Office of Renewable Energy Programs, Herndon, VA, OCS Study BOEM 2013-210, 23 pp., https://www.boem.gov/sites/default/files/environmental-stewardship/Environmental-Studies/Renewable-Energy/BOEM-final-report-on-Stakeholder-Info.pdf (last access: 28 April 2024), 2013.
Worzyk, T.: Submarine Power Cables: Design, Installation, Repair, Environmental Aspects, Power Systems, Springer Berlin, Heidelberg, 296 pp., https://doi.org/10.1007/978-3-642-01270-9, 2009.
Zhang, Y., Zhang, C., Chang, Y. C., Liu, W. H., and Zhang, Y.: Offshore wind farm in marine spatial planning and the stakeholders' engagement: Opportunities and challenges for Taiwan, Ocean Coast. Manage., 149, 69–80, 2017.