the Creative Commons Attribution 4.0 License.
the Creative Commons Attribution 4.0 License.
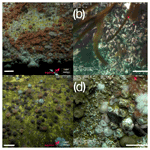
Artificial hard-substrate colonisation in the offshore Hywind Scotland Pilot Park
Malin Tivefälth
Iris Duranović
Svante Martinsson
Ane Kjølhamar
Kari Mette Murvoll
Artificial substrates associated with renewable offshore energy infrastructure, such as floating offshore wind farms, enable the establishment of benthic communities with a taxonomic composition similar to that of naturally occurring rocky intertidal habitats. The size of the biodiversity impact and the structural changes in benthic habitats will depend on the selected locations. The aim of the study is to assess colonisation and zonation, quantify diversity and abundance, and identify any non-indigenous species present within the wind farm area, as well as to describe changes in the epifouling growth between 2018 and 2020, with regards to coverage and thickness. This article is based on work undertaken within the offshore floating Hywind Scotland Pilot Park, the first floating offshore wind park established in the world, located approximately 25 km east of Peterhead, Scotland. The floating pilot park is situated in water depths of approximately 120 m, with a seabed characterised predominantly by sand and gravel substrates with occasional patches of mixed sediments. The study utilised a work class remotely operated vehicle with a mounted high-definition video camera, deployed from the survey vessel M/V Stril Explorer. A total of 41 structures, as well as their associated sub-components, including turbines substructures, mooring lines, suction anchors and infield cables, were analysed with regards to diversity, abundance, colonisation, coverage and zonation. This approach provides comprehensive coverage of whole structures in a safe and time-saving manner. A total of 11 phyla with 121 different taxa were observed, with macrofauna as well as macroalgae and filamentous algae being identified on the different structures. The submerged turbines measured approximately 80 m in height and exhibited distinct patterns of zonation. Plumose anemones (Metridium senile) and tube-building fan worms (Spirobranchus sp.) dominated the bottom and mid-sections (80–20 m) of the turbines, while kelp and other Phaeophyceae with blue mussels (Mytilus spp.) dominated top sections of the turbines (20–0 m). A general increase in the coverage of the epifouling growth between 2018 and 2020 was observed, whereas the change in thickness between years was more variable.
- Article
(8097 KB) - Full-text XML
-
Supplement
(420 KB) - BibTeX
- EndNote
The effects on local benthic habitats during installation works and operations of offshore wind farms (OWFs) are of a complex nature and extend both below and above the surface of the sea. Previous studies have shown that OWFs can impact areas through the introduction and spread of alien species (De Mesel et al., 2015; Wilhelmsson and Malm, 2008), affect organic matter deposition (De Borger et al., 2021) and carbon assimilation (Mavraki et al., 2020), and alter community structures (Coates et al., 2014; Degraer et al., 2020; Hutchison et al., 2020; Wilhelmsson and Malm, 2008) through the loss of soft-sediment habitats and the subsequent introduction of artificial hard-bottom substrates. The newly created habitat is usually larger than the lost habitat (Wilson and Elliott, 2009). The recorded impacts also include the recovery of the benthic biodiversity as a result of reduced trawling activities (Bergman et al., 2015; Coates et al., 2016) as well as an increase in nurseries for commercially important and/or protected species (Krone et al., 2017). The submerged structures (turbines and sub-components on the seabed) introduce hard substrates into areas in which there were formerly lacking, thus facilitating colonisation.
Studies conducted at OWFs around the North Sea show that the faunal and floral communities on turbines can further be categorised into distinct zones from the splash zone to the intertidal and deep subtidal zone (Degraer et al., 2020; De Mesel et al., 2015). These communities tend to develop over time (typically 5 to 6 years from the initial settling of organisms to reach the climax stage; Degraer et al., 2020) and evolve in characteristics, progressing from a pioneer stage (years 1 and 2) with sparse colonising taxa to an intermediate stage (years 3 to 5) exhibiting higher diversity followed by the final climax stage (from year 6 and onward) which is dominated by mussels, anemones and algae. The time taken to reach this final stage is dependent upon the foundation type (Degraer et al., 2019).
Global primary energy production has seen a 21 % increase in consumption between 2009 and 2019, where electricity from renewable sources, as of 2019, comprises 5 % of the total consumed primary energy (BP, 2020). Conventional wind farms are generally confined to shallow coastal waters (< 60 m) by technical and engineering constraints. Floating offshore wind farms (FOWFs), not being limited by these parameters, open up new possibilities with regards to installation locations.
Aim
Floating offshore wind farms (FOWFs), in contrast to most traditional OWFs, are to be located in deeper waters, at greater distances from the coast and other naturally occurring hard-bottom habitats not located on the seabed. Therefore, the aim of this study was to (1) ascertain whether or not impacts similar, with regards to colonisation on turbines and associated structures, to those observed at traditional OWFs were present at the Hywind Scotland Pilot Park; (2) assess if any zonation patterns were present on the Hywind Scotland Pilot Parks structures, similar to those observed at traditional OWFs; (3) to quantify diversity and abundances; and (4) identify if any non-indigenous species were present.
2.1 Study area
The world's first commercial floating offshore wind farm (FOWF), the Hywind Scotland Pilot Park, was constructed in 2017 and became operational the same year. The FOWF is located approximately 25 km east of Peterhead on the Scottish eastern coast and consists of five turbines, located at water depths of 100 to 130 m. The seabed comprises mainly sand and gravel substrates with mega ripples and occasional boulder fields classified as mixed sediments (Fig. 1).
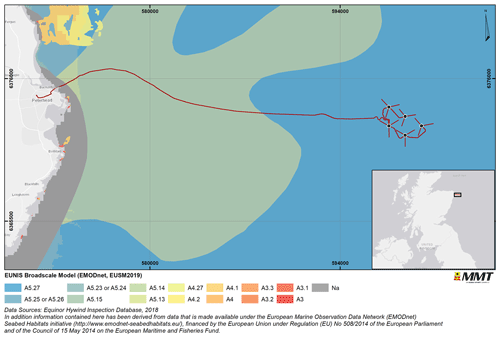
Figure 1Overview of the survey area and habitat according to the EUNIS (European Union Nature Information System; EUSM2019: EUSeaMap 2019) classification. The main habitat found in the survey area is A5.27 (deep circalittoral sand). Other habitats found are A5.25 (circalittoral fine sand), A5.26 (circalittoral muddy sand), A5.23 (infralittoral fine sand), A5.24 (infralittoral muddy sand), A5.15 (deep circalittoral coarse sediment), A5.14 (circalittoral coarse sediment), A5.13 (infralittoral coarse sediment), A4.27 (faunal communities on deep moderate-energy circalittoral rock), A4.2 (Atlantic and Mediterranean moderate-energy circalittoral rock), A4.1 (Atlantic and Mediterranean high-energy circalittoral rock), A4 (circalittoral rock and other hard substrata), A3.3 (Atlantic and Mediterranean low-energy infralittoral rock), A3.2 (Atlantic and Mediterranean moderate-energy infralittoral rock), A3.1 (Atlantic and Mediterranean high-energy infralittoral rock), A3 (infralittoral rock and other hard substrata) and Na (not applicable). Basemap sources: © OpenStreetMap contributors 2021. Distributed under the Open Data Commons Open Database License (ODbL) v1.0.
Unlike conventional, non-floating turbines whose foundations are secured directly to the seabed, the floating turbines are attached to the seabed using three suction anchors attached to the turbine substructure by heavy chains. The turbine substructures extend approximately 80 m below the sea surface, acting as a pendulum to keep the structure steady.
2.2 Data collection
The environmental survey was performed in collaboration with Reach Subsea and occurred simultaneously with a recurring structural inspection of the Hywind Scotland Pilot Park in June 2020. Video footage was obtained using a high-definition colour camera attached to a work class remotely operated vehicle (WROV) supported by LED floodlights and spotlights. Two lasers were positioned 10 cm apart. The WROV maintained a survey speed of 0.3 kn (0.6 km h−1). Video footage was recorded during the entire structural inspection of the turbine substructures, mooring lines, suction anchors and infield cables (Fig. 2). Additional video footage, solely for the environmental survey, was collected for turbine substructures HS01, HS02 and HS04 and infield cables HS04 to HS05 (QA01), HS01 to HS04 (QA02), HS02 to HS03 (QA04), and HS03 to HS05 (QA05), as well as the protective concrete mattress located on top of the QA01 cable (Fig. 3).
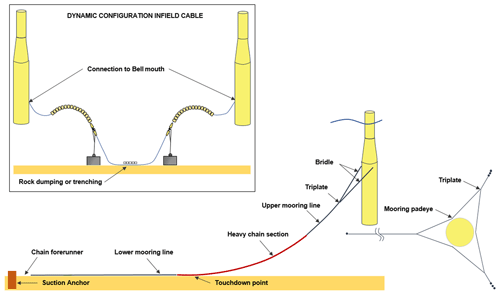
Figure 2Layout of turbine substructures, mooring lines, suction anchors and infield cables. Figure based on schematic provided by Equinor.
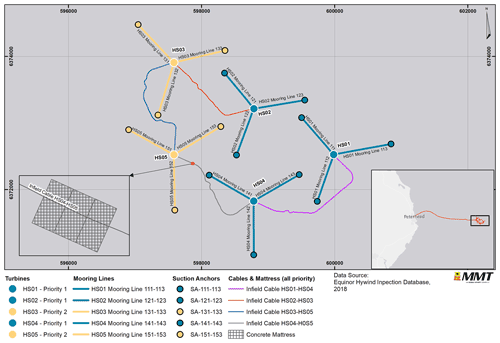
Figure 3Overview of survey area and priority and non-priority structures. Basemap sources: © OpenStreetMap contributors 2021. Distributed under the Open Data Commons Open Database License (ODbL) v1.0.
The three priority structures (HS01, HS02 and HS04) were investigated at a reduced speed of 0.2 kn (0.4 km h−1), and at three sides (12 o'clock (north), 4 o'clock and 8 o'clock) of the turbine substructures. In contrast, non-priority structures HS03 and HS05 were investigated simultaneously with the structural inspection. The priority structures were investigated from top to bottom at a closer distance compared to the rest of the survey. A distance of approximately 0.5 m was maintained throughout the majority of the environmental survey, and areas of interest were investigated at closer distances (< 0.3 m). Occasionally, when the sea state or obstructions occurred, the distance to the structure was increased up to approximately 1 m. The live feed from the WROV was monitored by one of the marine biologists on shift. This approach allowed for the fauna/areas of interest to be examined in closer detail if required.
2.3 Analysis methodology
The analyses of the acquired video data were performed in two steps. The first step was analysed in real time, from the live video feed from the WROV, and included documenting zonation, initial coverage estimates and common species, which were registered into a field log template in Microsoft Word. During the second step, the video was played back using VLC (VideoLAN Client) media player and comprised quality control of the field logs as well as the enumeration of individuals and assessment of percentage coverage of epifouling species. Lastly, the data were summarised into species lists, with separate lists for each structure and component.
Fauna was identified to the most detailed taxonomic level possible, mainly species, and counted or noted as present in the case of epifouling faunal (colonial and non-colonial) and floral species. This included the phyla Annelida, Bryozoa, Chlorophyta, Cnidaria, Phaeophyceae, Porifera and Rhodophyta, as well as fish, Sessilia, tunicates and bivalves. When a species could not be identified with certainty, the specimen was grouped into the nearest identifiable taxon of a higher rank, i.e. genus, family, order, etc. Overall coverage of epifouling taxa was quantified, as coverage for individual taxa proved problematic due to different taxa frequently co-habiting on the same spot.
Eggs (from cephalopods, nudibranchs and gastropods) identified during the survey were excluded from statistical analysis. Asteroidea and sea urchins were occasionally present in such abundance that it was difficult to count each individual, resulting in a likely underestimation of abundance.
2.3.1 Additional analyses
Data collected by Reach Subsea during the visual inspections of the structures in October–November 2018 and June 2020 were compiled, and changes in faunal coverage and thickness were compared. The 2018 survey was carried out using similar techniques with the exception of the additional data collected for the environmental survey in 2020, as mentioned in Sect. 2.2. The visual inspection in 2018 was not supported by marine biologists, and species were not recorded but rather growth, shape and in some cases phylum/order were, whereas the 2020 inspection was aided by marine biologists. To make the two datasets comparable, the data collected by the structural inspectors in 2018 and 2020 were compared.
Known references in the video footage, such as the dimensions of different components, were used to estimate the growth thickness. During the 2020 survey, the addition of parallel lasers spaced 10 cm apart further aided the assessment. Faunal and floral growth was observed for all different components and structures of the wind turbines by Reach Subsea structural inspectors and divided into hard (bivalves, poriferans, barnacles and tubeworms) and soft growth (bryozoans, hydroids, tunicates, cnidarians and macroalgae). In this paper, data have been grouped into the three main parts, turbine substructures, mooring lines and suction anchors, and differences between years were statistically tested using two-tailed paired t tests in Excel. Structures and sub-components not reported on during either the 2018 or the 2020 campaign have been excluded in this comparison. In total, 23 turbine sub-components (all included in turbine substructures), 125 mooring line sections and 15 suction anchors were inspected both years and included in the analyses. Gains and losses of broad groups between the years were noted and used to detect possible succession.
3.1 Identified species
The analyses of data from the Hywind Scotland Pilot Park yielded a total of 11 phyla, with 121 different taxa; 48 taxa were identified to be epifouling fauna, and 73 were identified as mobile taxa. In total an estimated number of 15 997 individuals were recorded during the analyses of the survey data (Tables 1 and S1 in the Supplement). The most abundant mobile taxon was Asteroidea, likely the common sea star (Asterias rubens), followed by small sea urchins (Psammechinus miliaris and/or Strongylocentrotus droebachiensis). Different species of crustaceans were present within the whole survey area and represented the dominating mobile phylum on the seabed. Three possible young colonies of deep-water coral (Desmophyllum pertusum, previously Lophelia pertusa) were identified along the infield cable between turbines HS01 and HS04. The colony identified at QA02–HS01 buoyancy modules at a depth of 73.5 m (Fig. 4) measured about 20 cm in diameter.
No invasive or non-indigenous species were identified during the 2020 survey (Scottish Natural Heritage, 2017). However, it should be noted that the use of a WROV without any physical sampling limits the ability to identify smaller species and identify certain filamentous species of red and brown algae.
Species observed on the seabed in close proximity to the structures included different crustaceans (the brown crab, C. pagurus; the Norway king crab, Lithodes maja; different species of squat lobsters; and a few individuals of lobster, Homarus spp.). Demersal fish, including different species of flatfish (Pleuronectiformes), haddock (Melanogrammus aeglefinus) and ling (Molva molva) were also found in high abundances around the structures. Squids, octopuses and rays were also observed.
3.2 Turbine substructures
The coverage of epifouling taxa was found to be high (∼ 80 % to 100 %), predominantly comprising the species Metridium senile and Spirobranchus sp. across the majority of the turbine surfaces (Fig. 5). The lower intertidal depths were dominated by blue mussels (Mytilus spp.) and brown algae. Mobile taxa present in high abundances included Echinidea, Asteroidea and Galatheoidea. Squat lobsters were generally noted below 40 m, while grazers such as sea urchins, sea stars and nudibranchs including Aeolidia papillosa were found all over the turbine substructures (Fig. 5). Sea urchins and sea stars occurred at all depths but were most abundant between 10 and 25 m, whereas nudibranchs were more abundant below 40 m.
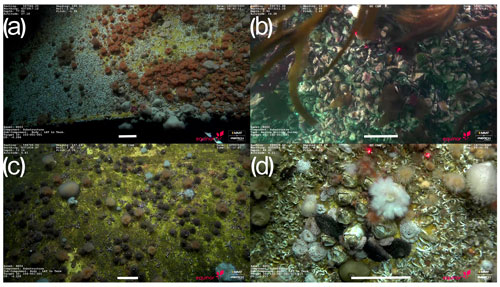
Figure 5Example of epifouling colonisation on turbine substructures. (a) Spirobranchus sp. and M. senile at the bottom of the HS03 substructure. (b) Substructure HS02, with Mytilus spp., Laminaria sp. and potential amphipod tubes at 3 m depth. (c) Substructure HS04, with grazing sea urchins and biofilm at 11 m depth. (d) Substructure HS01, with nudibranchs (A. papillosa) and barnacles (Balanoidea) at 48 m depth. Scale bar: 10 cm.
All turbine substructures were further assessed with regards to zonation and faunal composition. The estimated vertical zonation is illustrated in Fig. 6, with the top of the figure representing the sea surface at 0 m extending down to a depth of approximately 77 m representing the bottom of the turbine substructure. Four distinct faunal zones were identified at HS01, while HS02–HS05 comprised five different faunal zones. Turbine substructure HS01 comprised M. senile (50 %) and Spirobranchus sp. (50 %) from approximately 30 m to 77 m. At turbine substructure HS03, a change in the dominating species occurred at approximately 45 m and lower, where Spirobranchus sp. was noted to dominate completely. This pattern was also noted for turbine substructures HS02, HS04 and HS05 between 60 and 77 m. Species composition between 4 and 15 m below the surface differed between the five turbine substructures. Turbine substructure HS01 was colonised by a veneer of biofilm and Phaeophyceae; HS02 was colonised by M. senile and Laminaria sp.; HS03 was colonised by Laminaria sp. and other Phaeophyceae; HS04 was colonised by M. senile, Spirobranchus sp. and biofilm; and HS05 was dominated by M. senile, biofilm and Phaeophyceae. At turbine substructures HS01, HS02 and HS03, Mytilus spp. and Laminaria sp. were the dominating taxa from 0 m to approximately 4 m, and at HS04 and HS05, Mytilus spp. and different species of Phaeophyceae were dominant. Potential amphipod tubes could be observed in between the Mytilus spp. located close to the surface.
3.3 Suction anchors
There were no substantial differences between the epifouling communities on suction anchors associated with individual turbine substructures or between the different turbine groups. Each suction anchor was inspected along the top of the structures and separately around the sides. Different hydroids, predominantly Nemertesia ramosa and Ectopleura larynx, dominated the top of the suction anchors with coverage ranging from 20 % to 80 %. Spirobranchus sp. and E. larynx, with patches of barnacles, dominated the sides of the suction anchors with coverage from 60 % to 90 %. Mobile fauna such as Galatheoidea, Cancer pagurus, Palaemonidae, Lithodes maja and nudibranchs were frequently observed.
3.4 Mooring lines
No clear differences were noted on the mooring lines between the turbine substructures, but distinct zonation patterns were observed from top to bottom. The top chain was almost entirely covered by Balanoidea, M. senile and E. larynx, with an overall coverage ranging from 60 % to 100 %. The upper-middle chains were similar to the top chains, although the epifouling decreased as the chains descended towards the seabed with an overall coverage from 40 % to 80 %. The lowest parts of the chains, closest to and on top of the seabed, were dominated by crusts of Sabellaria spinulosa and E. larynx with coverage ranging from 80 % to 100 %. The mooring lines were estimated to have 100 % coverage or close to 100 %, and the composition of the middle chain was similar for all five turbine areas. Mobile fauna found on and adjacent to the mooring lines were A. rubens, Galatheoidea, C. pagurus, L. maja and Paguridae. An example of the colonisation along a typical mooring line (mooring line 111 of turbine HS01) is presented in Fig. 7, from top to bottom. The top chain was estimated to have an overall coverage between 60 % and 95 %, with an abundance of M. senile.
3.5 Infield cables and concrete mattress
From the bell mouth to touchdown, the overall dominating species was barnacle (Balanoidea), present abundantly along all four infield cables. Infield cables QA01 and QA02 comprised an overall faunal coverage of 100 % from each bell mouth to touchdown, whereas QA04 and QA05 comprised areas with lower faunal coverage. The infield cables were buried between each touchdown, and no faunal colonisation was therefore present.
The concrete mattress, located on top of QA01, was predominantly buried, and the overall faunal coverage was 40 %. The dominating species were S. spinulosa and E. larynx. Other epifouling fauna present included other hydroids such as N. ramosa, Tubularia indivisa and Urticina sp. Mobile fauna observed on the structure included Asteroidea, Galatheoidea, Paguridae, L. maja and C. pagurus. One individual of Pleuronectiformes, Homarus sp. and M. molva was present on the concrete mattress.
3.6 Comparison of faunal growth
Data from the 2018 inspection campaign, provided by Reach Subsea, were compared to the data acquired during the 2020 campaign (Table 2, Fig. 8). The coverage on the turbine substructures was not significantly different between the years, neither for the hard (p= 0.82) nor for the soft growth (p= 0.11). However, there was a significant decrease in the thickness of hard growth (p < 0.001), whereas the soft growth increased in thickness (p= 0.01). The coverage on the suction anchors increased in 2020 compared to 2018, both for the hard growth (p= 0.002) and soft growth (< 0.001); whereas the thickness of the cover decreased, the change was significant for the hard growth (p < 0.001) but not for the soft growth (p=0.10). For the mooring lines the coverage increased significantly both for the hard growth (p < 0.001) and the soft growth (p< 0.001). However, there were no significant changes in the thickness of the growth.
On the turbine substructures, the largest shift in composition was a loss of hydroids on 15 of 23 sub-components, and 7 sub-components had a gain of macroalgae. On the mooring lines, there was a loss of hydroids on 61 of 125 sub-components, a loss of tubeworms on 49 sub-components and a loss of barnacles on 45 sub-components.
Table 2Comparison of mean coverage and thickness of epifouling growth on turbine substructures, suction anchors and mooring lines between 2018 and 2020.
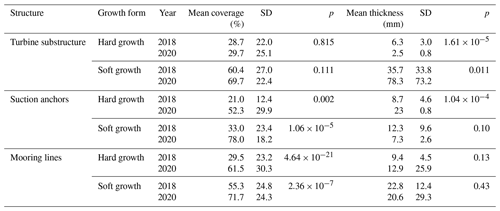
4.1 Identification of species
The data used in this study were collected from video footage using a WROV. The resolution and quality of the footage limit the detection and identification of smaller organisms, but it is more than sufficient for the detection and identification of larger organisms. Similar footage has been used successfully in other studies of fauna on offshore structures in the North Sea (e.g. Schutter et al., 2019). However, due to the limit in identifying smaller organisms to a lower level (e.g. species), species diversity and richness will be underestimated (Schutter et al., 2019).
The non-native American lobster (Homarus americanus) has been reported from the North Sea and the British Isles (Stebbing et al., 2012). Thus, it cannot with certainty be determined whether any of the lobsters observed during the current survey were H. americanus. Homarus gammarus and H. americanus are differentiated morphologically by the absence or presence of spines on the rostrum and are therefore difficult to distinguish without a physical specimen. Hybridisation between these species has also been recorded.
The barnacles observed on the structures were difficult to identify to the species level and are grouped in the superfamily Balanoidea. Two possible species have been considered, Balanus crenatus and Chirona hameri. External experts were consulted, and C. hameri was considered to be the probable species, but B. crenatus cannot be excluded without a physical sample.
The mooring lines and suction anchors on the seabed surface have provided additional opportunities for settling and colonisation by S. spinulosa, which was identified in the area during previous surveys (MMT, 2013). As the species occurs naturally in the area, the facilitated establishment created by the structures for S. spinulosa should not have a negative impact on the habitat. S. spinulosa habitats are often associated with high faunal biodiversity (Pearce et al., 2014), which creates feeding grounds for different species of fish.
The shape of the colony tentatively identified as deep-water coral (D. pertusum) is atypical for the species; however, similar dome-shaped colonies have been recorded on oil platforms in the North Sea (e.g. Gass and Roberts, 2006). Advised experts agree that the colony is likely D. pertusum, but due to the small size and uncharacteristic appearance, a positive identification would require close-up imagery of the calyx using a stills camera. Desmophyllum pertusum has not previously been recorded in this area, although colonies have been observed on offshore structures in the North Sea (Roberts, 2002; Bergmark and Jørgensen, 2014). Further, cold-water coral reefs also occur naturally on the continental shelf of western Scotland in water depths of 130 to 2000 m (Marine Scotland, 2016). Simulations of larval dispersal of D. pertusum from offshore structures in the North Sea demonstrate that there is potential for larvae to settle in the survey area (Henry et al., 2018).
4.2 Epifouling colonisation and dominant species
The high abundance of M. senile is consistent with findings from offshore structures in the North Sea (Whomersley and Picken, 2003; Kerckhof et al., 2012; De Mesel et al., 2015; Kerckhof et al., 2019). Species of the amphipod Jassa spp. have previously been identified as one of the dominating species on offshore structures in the North Sea with anemones and hydroids (Lindeboom et al., 2011; Krone et al., 2013) but were not observed during the current survey. The brown matter observed between the blue mussels could be amphipod tubes, such as Jassa spp., but a physical sample would be required to confirm this.
The epifouling community differed between the different structures with regard to species diversity. The painted turbine substructures harboured fewer taxa compared to the uncoated mooring lines. The tube-building worm (Spirobranchus sp.) and the anemone (M. senile) dominated the painted turbine substructures, while Balanoidea together with hydroids dominated the uncoated structures. Uncoated structures have been noted to comprise more diverse communities than steel monopiles (Kerckhof et al., 2012).
The concrete mattress was partially covered by sediment and is likely to be completely buried in the future. The structure provides a hard substrate for epifouling taxa, including hydroids and S. spinulosa. Several mobile taxa were observed, such as lobster, squat lobsters, flatfishes and ling. Should the structure remain exposed, it could continue to provide a suitable habitat for commercially important species and possibly maintain a S. spinulosa reef in the area.
4.3 Zonation
A depth zonation similar to, in regard to species composition and distribution, other offshore structures in the North Sea (Whomersley and Picken, 2003; Lengkeek and Bouma, 2009; De Mesel et al., 2015) was noted within the current survey area. Due to safety restrictions concerning close approaches to the turbine substructures, estimating the epifouling above the sea surface was not possible. The low intertidal zone was dominated by Mytilus spp., which was in line with previous studies conducted in the North Sea (Krone et al., 2013; Bergström et al., 2014). The deep subtidal zone extended from 10 m to 15 m below the surface and continued down to the bottom. From the low intertidal zone to approximately 25 m depth, there was generally a high presence of biofilm and fewer epifouling species, which could be due to grazing fauna that were occasionally numerous.
Four depth zonations were observed at turbine substructure HS01, and five were at substructures HS02 to HS05. Turbine substructure HS01 lacked the deepest Spirobranchus sp.-dominated zonation found at the other four substructures. The difference is likely due to local variation and faunal spread. The differences were not clear enough to indicate whether or not the currents or the distance to the shore would affect the zonation and growth of epifaunal species. The zonation noted along the mooring lines comprised a different species community than those identified at the turbine substructures. The mooring lines were generally dominated by M. senile and Balanoidea at the same water depths as where the turbine substructures were dominated by Spirobranchus sp. and M. senile. The top and upper-middle sections of the mooring lines were dominated by M. senile and Balanoidea. The middle chain comprised, overall, lower faunal colonisation.
4.4 Comparison of faunal growth
Coverage of both hard and soft growth has significantly increased from 2018 to 2020 on both suction anchors and mooring lines but not on the turbine substructures. The change in thickness is more variable compared to coverage, with a significant decrease of hard growth noted on both the turbine substructures and suction anchors, while an increase of the soft-growth thickness was observed on the turbine substructures. Large standard deviations were observed for many of the measurements, due to the high variation between the structures. Further, the lack of lasers during the 2018 survey may have contributed to the variation of the measurements between the years.
4.5 Succession
The gain and loss of taxa observed indicates a shift in taxonomic composition between 2018 and 2020, with mainly a decrease in hydroids, tubeworms and barnacles; this was corroborated in discussions with the survey team who performed the initial visual inspection in 2018, and they confirmed that faunal composition had changed between the 2 years, indicating a succession. The observed changes seem to follow the same trend regarding succession stages that has previously been observed on offshore installations in the North Sea (Rumes et al., 2013; Whomersley and Picken, 2003); tubeworms and hydroids have been reported as the first to colonise the structures, followed by M. senile and Alcyonium digitatum, who outcompeted the early colonisers by overgrowing. This seems to be the case at Hywind FOWF, which would indicate that the park is currently in the species-rich intermediate stage, moving towards a more M. senile-dominated stage with less biodiversity. The taxonomical resolution in the data collected in 2018 limits the analysis of succession between the years. As in previous studies in the North Sea (De Mesel et al., 2015; Whomersley and Picken, 2003), a zonation was established just a few years after the installation of the structures. Echinoderms were present in high abundance and are considered an important grazer that affects the epifouling community (Witman, 1985) and could keep the epifouling colonisation growth suppressed.
Species characterisation during visual inspection gave a good overview of the survey area and the higher phyletic community composition. The species detail level was limited when fauna was small and/or the environmental conditions (i.e. strong currents, poor weather, etc.) were poor. To confirm the presence or absence of invasive and non-indigenous species on the structures, physical samples are recommended for future surveys as a complement to the visual inspection. Overall, the approach provides comprehensive coverage of whole structures in a safe and time-saving manner.
The epifouling fauna and flora identified were all species naturally occurring in Scottish waters and around the North Sea. However, the community structure, with its high abundances of M. senile, is different when comparing the structures to that which is generally observed on rocky intertidal habitats. Metridium senile, Spirobranchus sp., M. edulis and barnacles are predominant species typically observed on artificial structures in UK waters and seem to take advantage of newly installed surfaces (Bessel, 2008).
Four mobile taxa featured on the Scottish Biodiversity List and as Priority Marine Features were identified in close proximity of the structures: Atlantic cod (Gadus morhua), ling (M. molva), sand eel (Ammodytes spp.) and whiting (Merlangius merlangus). The overall epifaunal colonisation was assessed to almost 100 % on the different structures, with some minor local variations noted. Epifouling colonisation observed during the survey showed overall similarities with the colonisation of other artificial structures in the North Sea regarding early colonisers and epifouling on structures.
The list of taxa found on the structures is available in Supplement Table S1. The full dataset, consisting of video files, is too large to upload but is available upon request.
The supplement related to this article is available online at: https://doi.org/10.5194/wes-7-801-2022-supplement.
AK and KMM together funded the project, conceptualised the survey and reviewed the manuscript. The survey was carried out by RK and MT. Methodology, data analysis and the manuscript draft were equally contributed to by RK, MT and ID. SM aided in the revision of the manuscript and performed the statistical analyses.
Authors Rikard Karlsson, Malin Tivefälth and Iris Duranović were contracted as consultants by Equinor with the aim of writing a scientific paper regarding the findings of the 2020 Hywind Scotland Pilot Park inspection and the subsequent assessment of the impacts of floating offshore wind farms on marine life. The same authors had previously been contracted by Equinor to conduct the 2020 inspection survey of the Hywind Scotland Pilot Park as well as to analyse the collected data and compile an environmental survey report.
Publisher’s note: Copernicus Publications remains neutral with regard to jurisdictional claims in published maps and institutional affiliations.
The authors would like to thank Equinor for funding the survey and sharing these findings. The authors would also like to thank the ROV pilots and structure inspectors at Reach Subsea for their assistance as well as the crew on board the M/V Stril Explorer.
This research has been supported by Equinor (grant no. 4590209614).
This paper was edited by Jonas Teilmann and reviewed by Jørgen Hansen and Joop W. P. Coolen.
Bergman, M. J. N., Ubels, S. M., Duineveld, G. C. A., and Meesters, E. W. G.: Effects of a 5-year trawling ban on the local benthic community in a wind farm in the Dutch coastal zone, ICES J. Mar. Sci., 72, 962–972, https://doi.org/10.1093/icesjms/fsu193, 2015.
Bergmark, P. and Jørgensen, D.: Lophelia pertusa conservation in the North Sea using obsolete offshore structures as artificial reefs, Mar. Ecol.-Prog. Ser., 516, 275–280, https://doi.org/10.3354/meps10997, 2014.
Bergström, L., Kautsky, L., Malm, T., Rosenberg, R., Wahlberg, M., Capetillo, N. Å., and Wilhelmsson, D.: Effects of offshore wind farms on marine wildlife – a generalized impact assessment, Environ. Res. Lett., 9, 034012, https://doi.org/10.1088/1748-9326/9/3/034012, 2014.
Bessel, A.: Kentish Flats offshore wind farm turbine foundation faunal colonisation diving survey, Report no. 08/J/1/03/1034/0839, Tech. rep., Emu Ltd on behalf of Kentish Flats Ltd., 2008.
BP: Statistical Review of World Energy 2020, https://www.bp.com/content/dam/bp/business-sites/en/global/corporate/pdfs/energy-economics/statistical-review/bp-stats-review-2020-full-report.pdf (last access: 31 March 2022), 2020. Petroleum, B. BP Statistical Review of World Energy 2020, 2020. Please see link:
Coates, D. A., Deschutter, Y., Vincx, M., and Vanaverbeke, J.: Enrichment and shifts in macrobenthic assemblages in an offshore wind farm area in the Belgian part of the North Sea, Mar. Environ. Res., 95, 1–12, https://doi.org/10.1016/j.marenvres.2013.12.008, 2014.
Coates, D. A., Kapasakali, D.-A., Vincx, M., and Vanaverbeke, J.: Short-term effects of fishery exclusion in offshore wind farms on macrofaunal communities in the Belgian part of the North Sea, Fish. Res., 179, 131–138, https://doi.org/10.1016/j.fishres.2016.02.019, 2016.
De Borger, E., Ivanov, E., Capet, A., Braeckman, U., Vanaverbeke, J., Grégoire, M., and Soetaert, K.: Offshore Windfarm Footprint of Sediment Organic Matter Mineralization Processes, Front. Mar. Sci., 8, 632243, https://doi.org/10.3389/fmars.2021.632243, 2021.
Degraer, S., Brabant, R., Rumes, B., and Vigin, L. (Eds.): Environmental Impacts of Offshore Wind Farms in the Belgian Part of the North Sea: Marking a Decade of Monitoring, Research and Innovation, Brussels: Royal Belgian Institute of Natural Sciences, OD Natural Environment, Marine Ecology and Management, 134 pp., 2019.
Degraer, S., Carey, D., Coolen, J. W. P., Hutchison, Z., Kerckhof, F., Rumes, B., and Vanaverbeke, J.: Offshore Wind Farm Artificial Reefs Affect Ecosystem Structure and Functioning: A Synthesis, Oceanography, 33, 48–57, https://doi.org/10.5670/oceanog.2020.405, 2020.
De Mesel, I., Kerckhof, F., Norro, A., Rumes, B., and Degraer, S.: Succession and seasonal dynamics of the epifauna community on offshore wind farm foundations and their role as stepping stones for non-indigenous species, Hydrobiologia, 756, 37–50, https://doi.org/10.1007/s10750-014-2157-1, 2015.
Gass, S. E. and Roberts, J. M.: The occurrence of the cold-water coral Lophelia pertusa (Scleractinia) on oil and gas platforms in the North Sea: colony growth, recruitment and environmental controls on distribution, Mar. Pollut. Bull., 52, 549–559, https://doi.org/10.1016/j.marpolbul.2005.10.002, 2006.
Henry, L. A., Mayorga-Adame, C. G., Fox, A. D., Polton, A. J., Ferris, J. S., McLellan, F., McCabe, C., Kutti, T., and Roberts, M.: Ocean sprawl facilitates dispersal and connectivity of protected species, Sci. Rep.-UK, 8, 11346, https://doi.org/10.1038/s41598-018-29575-4, 2018.
Hutchison, Z. L., LaFrance Bartley, M., Degraer, S., English, P., Khan, A., Livermore, J., Rumes, B., and King, J. W.: Offshore wind energy and benthic habitat changes: Lessons from Block Island Wind Farm, Oceanography, 33, 58–69, https://doi.org/10.5670/oceanog.2020.406, 2020.
Kerckhof, F., Rumes, B., Norro, A., Houziaux, J., and Degraer, S.: A comparison of the first stages of biofouling in two offshore wind farms in the Belgian part of the North Sea, in: Offshore wind farms in the Belgian part of the North Sea: Heading for an understanding of environmental impacts, edited by: Degraer, S., Brabant, R., and Rumes, B., Royal Belgian Institute of Natural Sciences, Management Unit of the North Sea Mathematical Models, Marine ecosystem management unit, Brussels, 17–39, 2012.
Kerckhof, F., Rumes, B., and Degraer, S.: About “Mytilisation” and “Slimeification”: A Decade of Succession of the Fouling Assemblages on Wind Turbines off the Belgian Coast, in Environmental Impacts of Offshore Wind Farms in the Belgian Part of the North Sea: Marking a Decade of Monitoring, Research and Innovation, edited by: Degraer, S., Brabant, R., Rumes, B., and Vigin, L., Royal Belgian Institute of Natural Sciences, OD Natural Environment, Marine Ecology and Management, Brussels, 73–84, ISBN 978-9-0732-4249-4, 2019.
Krone, R., Gutow, L., Joschko, T. J., and Schröder, A.: Epifauna dynamics at an offshore foundation–implications of future wind power farming in the North Sea, Mar. Environ. Res, 85, 1–12, https://doi.org/10.1016/j.marenvres.2012.12.004, 2013.
Krone, R., Dederer, G., Kanstinger, P., Krämer, P., Schneider, C., and Schmalenbach, I.: Mobile demersal megafauna at common offshore wind turbine foundations in the German Bight (North Sea) two years after deployment – increased production rate of Cancer pagurus, Mar. Environ. Res., 123, 53–61, https://doi.org/10.1016/j.marenvres.2016.11.011, 2017.
Lindeboom, H. J., Kouwenhoven, H. J., Bergman, M. J. N, Bouma, S., Brasseur, S., Daan, R., Fijn, R. C., de Haan, D., Dirksen, S., van Hal, R., Hille Ris Lambers, R., ter Hofstede, R., Krijgsveld, K. L., Leopold, M., and Scheidat, M.: Short-term ecological effects of an offshore wind farm in the Dutch coastal zone; a compilation, Environ. Res. Lett., 6, 035101, https://doi.org/10.1088/1748-9326/6/3/035101, 2011.
Lengkeek, W. and Bouma, S.: Development of underwater flora- and fauna communities on hard substrates of the offshore wind farm Egmond aan Zee (Report No. 08-220), Bureau Waardenburg bv., 2009.
Marine Scotland: Cold Water Coral Reef, http://marine.gov.scot/information/cold-water-coral-reef (last access: 17 September 2021), 2016.
Mavraki, N., Degraer, S., Vanaverbeke, J., and Braeckman, U.: Organic matter assimilation by hard substrate fauna in an offshore wind farm area: a pulse-chase study, ICES J. Mar. Sci., 77, 2681–2693, https://doi.org/10.1093/icesjms/fsaa133, 2020.
MMT: Environmental Survey Report Hywind Offshore Windfarm, Statoil, https://www.equinor.com/content/dam/statoil/documents/impact-assessment/Hywind/Statoil-Environmental survey report.pdf (last access: 31 March 2022), 2013.
Pearce, R., Fariñas-Franco, J. M., Wilson, C., Pitts, J., deBurgh, A., and Somerfield, P. J.: Repeated mapping of reefs constructed by Sabellaria spinulosa Leuckart 1849 at an offshore wind farm site, Cont. Shelf Res., 83, 3–13, https://doi.org/10.1016/j.csr.2014.02.003, 2014.
Roberts, J. M.: The occurrence of the coral Lophelia pertusa and other conspicuous epifauna around an oil platform in the North Sea, Underwater Technol., 25, 83–92, 2002.
Rumes, R., Coates, D., Demesel, I., Derweduwen, J., Kerckhof, F., Reubens, J., and Vandendriessche, S.: Does it really matter? Changes in species richness and biomass at different spatial scales, in: Environmental impacts of offshore wind farms in the Belgian part of the North Sea: Learning from the past to optimise future monitoring programmes, edited by: Degraer, S., Brabant, R., and Rumes, B., Royal Belgian Institute of Natural Sciences, Operational Directorate Natural Environment, Marine Ecology and Management Section, 239 pp., ISBN 9789090279282, 2013.
Schutter, M., Dorenbosch, M., Driessen, F. M. F., Lengkeek, W., Bos, O. G., and Coolen, J. W. P.: Oil and gas platforms as artificial substrates for epibenthic North Sea fauna: Effects of location and depth, J. Sea. Res., 153, 101782, https://doi.org/10.1016/j.seares.2019.101782, 2019.
Scottish Natural Heritage: Marine non-native species, https://www.nature.scot/professional-advice/land-and-sea-management/managing-coasts-and-seas/marine-non-native-species (last access: 17 September 2021), 2017.
Stebbing, P., Johnson, P., Delahunty, A., Clark, P. F., McCollin, T., Hale, C., and Clark, S.: Reports of American lobsters, Homarus americanus (H. Milne Edwards, 1837), in British waters, BioInvasions Records, 1, 17–23, https://doi.org/10.3391/bir.2012.1.1.04, 2012.
Whomersley, P. and Picken, G.: Long-term dynamics of fouling communities found on offshore installations in the North Sea, J. Mar. Biol. Assoc. UK., 83, 897–901, https://doi.org/10.1017/S0025315403008014h, 2003.
Wilhelmsson, D. and Malm, T.: Fouling assemblages on offshore wind power plants and adjacent substrata, Estuar. Coast. Shelf S., 79, 459–466, https://doi.org/10.1016/j.ecss.2008.04.020, 2008.
Wilson, J. C. and Elliott, M.: The Habitat-creation Potential of Offshore Wind Farms, Wind Energ., 12, 203–221, https://doi.org/10.1002/we.324, 2009.
Witman, J.: Refuges, biological disturbance, and rocky subtidal community structure in New England, Ecol. Monogr., 55, 421–445, https://doi.org/10.2307/2937130, 1985.